Research Article, J Plant Physiol Pathol Vol: 10 Issue: 5
Interactive Effects of Groundnut Rossette Disease Infection and Drought Stress on Growth and Performance of Groundnuts (Arachis Hypogaea L.)
Kwadwo Owusu Ayeh1*, Adoteye Emmanuella Grace1, Enu-Kwesi Lewis1 and Andrew Sarkodie Appiah2
1Department of Plant and Environmental Biology, School of Biological Sciences, University of Ghana-Legon, Ghana
2Biotechnology and Nuclear Agriculture Institute, Ghana Atomic Energy, Commission, Kwabenya-Ghana
*Corresponding Author: Kwadwo Owusu Ayeh, Department of Plant and Environmental Biology, School of Biological Sciences, University of Ghana-Legon, Ghana, E-mail: koayeh@ug.edu.gh
Received date: 29 April, 2022, Manuscript No. JPPP-22-57950;
Editor assigned date: 04 May, 2022, Pre QC No. JPPP-22-57950 (PQ);
Reviewed date: 13 May, 2022, QC No. JPPP-22-57950;
Revised date: 22 May, 2022, Manuscript No: JPPP-22-57950 (R);
Published date: 30 May, 2022, DOI: 10.4172/2329-955X.1000323
Citation: Ayeh KO, Grace AE, Lewis EK, Appiah AS (2022) Interactive Effects of Groundnut Rossette Disease Infection and Drought Stress on Growth and Performance of Groundnuts (Arachis Hypogaea L.). J Plant Physiol Pathol 10:5.
Abstract
Groundnut (Arachis hypogea L.) production is in Ghana is affected by both biotic and abiotic factors resulting in significant losses. However, research into the impact of combined biotic and abiotic stress factors on growth of groundnut, an important crop grown in Ghana is scanty. Our research sought to give a first insight into the combined and simultaneous effect of virus and water stress effect on growth, development and yield of groundnut. Four (4) accessions of groundnut: 18001; 18002; 18003 and Chinese obtained from Kanjo in the Northern region of Ghana were subjected to water and viral stress factors in a factorial screen house experiment. The accessions were grafted with infected plant material showing visible symptoms of the Groundnut Rosette Disease (GRD) and combined with 3 different watering regimes, namely: 2-days rehydration, 3-days rehydration and 5-days rehydration. The treated accessions were subsequently monitored for their various treatment combinations using physiological, morphological and biochemical parameters. The highest mean symptom severity value of 3.9 was recorded in the combined biotic and severe abiotic stress treatment as compared to symptom severity score of 1.5 in the control. Symptoms observed in combined virus and water stress treatments (severe) included shortening of internode length, chlorosis and reduced yield. Plant height and leaf area were found to have reduced in response to both abiotic and biotic stress factors. Crop yield in terms of mean number of pods per plant were low in the combined biotic and abiotic severe water treatment. Results of the current study highlight the effect of combined virus infection and drought on groundnut production and the need to breed for virus resistant and drought tolerant varieties to ensure sustainable production.
Keywords: Groundnut rosette disease; Grafting; Water stress; Relative water content; Disease incidence; Chlorophyll content
Introduction
Groundnut (Arachis hypogaea L.), commonly known as peanut in some parts of the world, is considered a nut from the Fabaceae (legume) family [1]. It is a nitrogen-fixing plant mainly grown for its edible seeds; and cultivated widely in the arid and semi-arid regions with erratic rainfall patterns and increasing ambient aerial and edaphic temperatures where water supply to the roots is limited; and transpiration rates are high [2].
Although groundnuts have great nutritional benefits, their production has been hampered by several factors including both biotic and abiotic stresses. A major biotic stress factor is the Groundnut Rosette Disease (GRD), which affects groundnuts and places diverse levels of constraints on groundnut production depending on its severity.The disease is known to be caused by the synergistic only the interaction between three agents namely, Groundnut Rosette Assistor Virus (GRAV), Groundnut Rosette Virus (GRV), and its Satellite RNA (SatRNA), which are all transmitted by the aphid, Aphis craccivora Koch [3,4]. Three forms of GRD namely chlorotic rosette, green rosette and mosaic rosette are known to exist with the green and mosaic rosette being the dominant type [5,6]. Initial symptoms of the GRD include chlorosis or dark greening of the lamina in emerging leaves whereas older leaves curl up. However, the exposure of plants to stresses in nature is not exclusive to biotic stress factors alone but rather a cumulation of abiotic stress factors such as water and salinity stresses which are known to have detrimental effects on plants leading to primary causes of crop loss globally [7,8]. It is therefore not unusual for plants to be exposed to concurrent environmental biotic and abiotic stress factors. Consequently, such plants perceive each of these biotic and abiotic environmental stresses separately and provide a measured response to each of them separately [9,10,11]. In addition, Suzuki et al, reported that when two or more stress factors that affect plants (specifically a major abiotic stress combined with a pathogen infection) occur simultaneously such as heat and bacterial infection or drought and viral infection, the response of plants to these combinations is unique and cannot be derived from the plant’s response to the individual application of each of the stresses.
To date, research on the simultaneous effect of two or more stress factors on groundnuts that cause constraints to their growth and development has not been given the needed attention. In a world where climate change has become a topical concern, groundnut production faces immense challenges which include cultivation in areas with erratic rainfall patterns and hence likely to be affected by water stress (drought) as well as effects of plant viruses that may contribute to poor growth that will affect the total production or output. In order to increase yield, it is important to understand the physiological responses to the interactive effect (s) of both water and viral stresses on groundnut production in order to ensure food security while meeting the nutritional needs of the people and improving the livelihood of groundnut farmers [12].
This study is most likely the first formal report that gives insights into the preliminary effects of both virus and water stress effects on the growth and yield of some groundnut accessions in Ghana.
Materials and Methods
Plant materials: The study was conducted using uniformly-sized cultivated groundnut seeds of four (4) accessions, namely: 18001, 18002, 18003, and Chinese obtained from Kanjo in the Northern region of Ghana.
Soil: Soil used in this study was obtained from the Agricultural Teaching Gardens at the University of Ghana. 40 g of soil was weighed and placed into a plastic bucket. A total of 192 buckets were used for the study.
Study site: The study was carried out in the screen house of the department of plant and environmental biology, university of Ghana.
Grafting: A total of seventy-two groundnut seeds (of the 4 selected cultivated groundnut accessions) were planted and arranged in 3 blocks, each block containing 24 seeds and arranged into 6 replicates for each of the four accessions obtained from Kanjo. After 2 weeks, four of the 6 germinated seeds of groundnuts were grafted with GRDinfected groundnut plants showing typical symptoms of the disease whereas two of the six replicates were grafted with healthy groundnut plant material to serve as controls. All experiments were carried out using Randomized Complete Block Design (RCBD) with 3 treatment blocks namely Normal (N), Moderate (M) and Severe (S).
Watering regime: During the first 4 weeks of the study prior to the commencement of the watering regime, all groundnut seedlings were saturated with water. Subsequently, the grafted groundnut seedlings of all the 4 different accessions were subjected to 3 different watering regimes. The watering regimes are as follows: In the first regime, plants were rehydrated every 2 days (defined as an absence of stress– normal), in the second and third regimes, plants were rehydrated every three days (defined as the moderate application of stress) and 5 days (severe application of water stress) respectively. Each potted plant was rehydrated with 300 ml of water on each watering day in the respective water regimes under each treatment block.
Disease incidence: The number of plants showing symptoms of the GRD after artificial inoculation by grafting was expressed as a percentage of the total number of plants in the study.
Symptom severity score: Individual plants were visually assessed and scored using a 0–5 scale (Table 1).
Table 1: Disease scale for rating of groundnut rosette disease.
Plant height: The heights of all plants in each treatment block were measured weekly for 9 weeks using a metre rule.
Chlorophyll content: The Spectral Absorbance Data (SPAD) chlorophyll content was measured in newly emerged leaves in each treatment block weekly for 8 weeks using the SPAD Chlorophyll Content Metre (CCM- 200 plus, Opti-Sciences Inc.).
Leaf area: Leaf area was measured on two emerging young leaves twelve (12) Weeks After Planting (WAP) using Leaf Area Meter (AM350, Serial no. 34303, ADC Bio Scientific Ltd)
Relative Water Content (RWC): RWC was measured 12 WAP using Barrs and Weatherly (1962) protocol for determination of RWC. Three leaves were detached from each groundnut plant and a cork borer with a diameter of 1.2 cm was used to create leaf disc in each leaf, placed in a petri dish and covered. The Fresh Weight (FW) of leaf discs was measured using a fine balance (AL 104; Mettler Toledo, Columbus, OH, USA). Leaf discs were subsequently floated in 10 ml of distilled water in a petri dish and covered for 3 hours after which they were removed and surface blotted with tissue paper. Leaf discs were weighed to determine their Turgid Weight (TW) after which they were dried in an oven at 60â?? for 24 hours and the Dry Weight (DW) determined. The RWC was then calculated as;
RWC=Fresh Weight (FW)-Dry Weight (DW)/ Turgid Weight (TW)-Dry Weight (DW) × 100
Soil Moisture Content (SMC): SMC was determined 12 WAP using the Gravimetric Method and expressed as a percentage of the initial soil weight. A cork borer with diameter of 1.2 cm was used to collect soil samples from a 5 cm radius along the sides of each plastic container and quickly emptied into petri dishes and covered. Collected soil samples were weighed using a fine balance (AL 104; Mettler Toledo, Columbus, OH, USA). The soil samples were further dried at 60â?? for 24hr in an oven. The dried soil samples were weighed to determine their dry weight. The SMC was then calculated as follows:
SMC=Initial Weight (IW)-Dry Weight (DW)/Initial Weight (IW)- Dry Weight (DW) × 100
Number of pods per plant: Harvested pods from accessions used in the respective water regimes in this study were counted and the mean value determined.
Mean seed weight: Mean seed weight was determined using 10 randomly selected seeds from each accession and in each treatment block. The process was repeated three times.
Root and shoot ratio: Weights of both root and shoot were taken separately and recorded. The root to shoot ratio (R/S) was calculated as;
R/S= Dry weight for root/ Dry weight for shoot
Data analysis: All data sets were analysed by one-way Analysis of Variance (ANOVA) and means compared using Tukey-pairwise comparison analysis with Minitab Software (v.17.0).
Results
Disease incidence: Mean disease incidence of accession 18003 was the highest (83.3%) with accession 18001 recording the lowest disease incidence of 50% across all treatment blocks. When disease incidence was considered within each treatment, accession 18003 had the highest disease incidence of 75% within the normal treatment with the other three accessions recording 50% disease incidence. Within the moderate treatment, 18002 had the lowest disease incidence with 50% whereas Chinese recorded the highest with a 100% disease incidence.
However, within the severe treatment, 18002 and 18003 recorded the highest disease incidence of 100% (Figure 1).
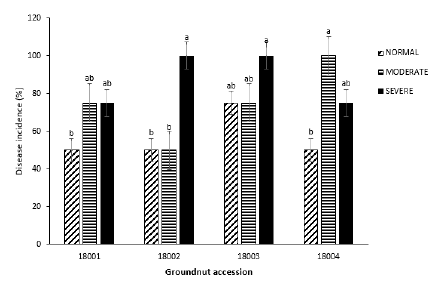
Figure 1: Mean GRD incidence on four groundnut accessions under three watering regimes 56 DAG.
Symptom severity: All groundnut accessions grafted with the infected groundnut material showed significant differences in severity score compared to the non-infected plants (P=0.00, F=190.22). Accession 18002 in severe block recorded the highest mean symptom severity score of 3.9 ± 0.2 in all three treatment blocks and was significantly different from all other accessions in all three rehydration blocks with 18001 recording the lowest mean symptom severity in the moderate rehydration block with a mean score of 1.8 ± 0.1. Across all three rehydration treatments, symptom severity scores were higher with increasing water stress levels (Figure 2).
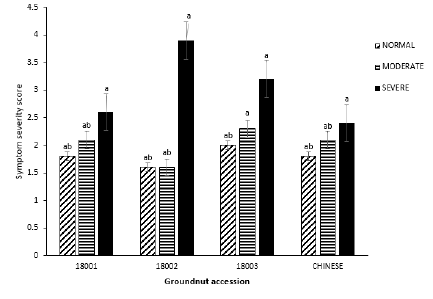
Figure 2: Mean symptom severity score of the four groundnut accessions under three watering regimes at 56 DAG.
Symptoms that were generally observed during the study included shortening of internodes (Figure 3A) as compared to normal internode length (Figure 3 B), chlorosis and reduced leaf sizes (Figure 3C) as against its corresponding normal plant (Figure 3D), leaf curling (Figure 3E), leaf puckering (Figure 3F) and stunted plant growth (Figure 3G) with its corresponding normal plant (Figure 3).
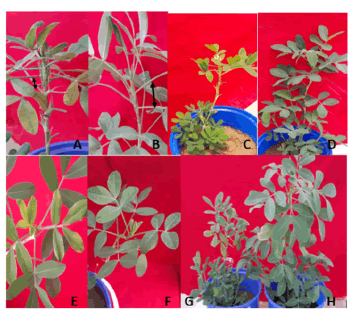
Figure 3: Virus symptoms observed in groundnut plants after grafting with GRD-infected scions. A, C, E, F and G are diseased plants. B, D and H are healthy plants.
Accessions 18002 and 18003 expressed typical GRD symptoms which included severe plant stunting, leaf chlorosis and severe reduction in leaf sizes in all treatment blocks as severity score increased with rising water stress levels. Cultivar Chinese expressed GRD symptoms with mild stunting and chlorosis while healthy plants of all cultivars were disease free and showed no symptoms of virus infection.
Plant height: Mean plant height for all the groundnut accessions in all three watering regime blocks were significantly different (P=0.042, F=1.84). Plant height was generally found to decrease with increasing drought levels (Figure 4) Virus infection had an effect on plant height as plant height reduced in infected groundnut plants when compared with their respective controls. Percentage height reduction was high in cultivars Chinese (36%) and 18001 (29.8%).
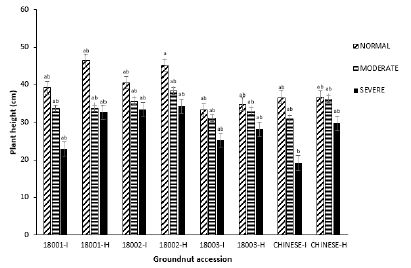
Figure 4: Mean plant heights of four groundnut accessions under three watering regimes.
Chlorophyll content: GRD infection had a significant (P=0.00, F=6.8) effect on chlorophyll content of the groundnut accessions when compared to their respective healthy controls. Chlorophyll content decreased with increasing water stress levels although this was not observed in infected plants of cultivar 18003 (Figure 5). Infected plants had lower chlorophyll content when compared to their respective healthy control plants which recorded higher chlorophyll content. Within the normal block, accessions 18001 and 18002 recorded the highest (28.6 μmol/m2) and lowest (24.0 μmol/m2) chlorophyll content respectively among the infected plants. In the moderate watering regime, accession Chinese had the highest chlorophyll content (19.1 μmol/m2) whilst 18003 recorded the least (17.1 μmol/m2). In the severe water treatment, infected plants of accessions 18003 and 18002 had the highest (17.9 μmol/m2) and the lowest (14.1 μmol/m2) chlorophyll content respectively.
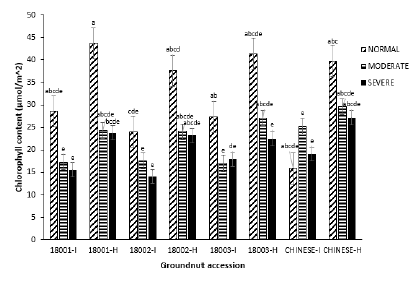
Figure 5: Mean chlorophyll content of four groundnut accessions under three watering regimes.
Leaf area: Leaf area decreased with increasing stress levels in infected plants compared to their healthy counterparts in all watering regime blocks. Accessions 18001, 18002 and 18003 recorded the highest leaf areas for the normal (91.5 ± 1.9 cm2), moderate (84.0 ± 2.3 cm2) and severe (65.6 ± 1.6 cm2) blocks respectively. Accession Chinese had the lowest leaf area in normal block (80.9 ± 1.2 cm2) as well as the moderate block (69.4 ± 2.1 cm2). However, 18002 recorded the lowest leaf area in the severe block (64.7 ± 1.95 cm2) (Figure 6), with 32.2% reduction in leaf area when compared to healthy controls.
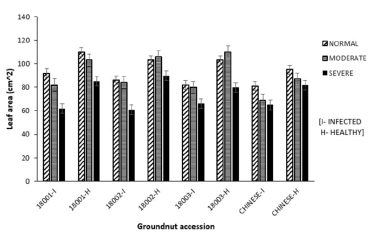
Figure 6: Mean leaf area of four groundnut accessions under three watering regimes.
Relative Water Content (RWC): Mean RWC values for the three watering regime blocks were significantly different from each other (P=0.003, I=5.46) with the normal block recording the highest RWC values followed by moderate treatment and the least being the severe treatment block.
Generally, the groundnut accessions had significant influence on RWC values (P=0.00, F=446.53) with the highest RWC value in the normal block recorded in 18003 (83.9 ± 0.3%) and the lowest (65.2 ± 0.9%) in 18002. In the moderate block, accessions 18003 and 18002 recorded the highest RWC values of 66.6 ± 0.5% (highest) and 41.0 ± 0.01% (lowest) lowest respectively. Chinese had the highest RWC value (53.2 ± 0.4%) within the severe block whereas 18002 had the lowest value (24.5 ± 0.9%) (Table 2).
Table 2: Relative water content of the four groundnut accessions under the three watering regimes.
Soil Moisture Content (SMC): The effect of groundnut accessions on soil moisture content was significant (P=0.00, F=71.61). SMC values recorded were higher for the normal block and decreased gradually as drought stress levels increased (Table 3).
Table 3: Soil moisture content of four groundnut accessions under three watering regimes
Number of pods: Total number of pods varied significantly within treatments and across treatments in each groundnut accession (P=0.00, F=71.61). Within the normal treatment, there was no significant effect on the mean number of harvested pods (P> 0.05, F=2.71). Infected plants within this block had lower pod numbers than healthy plants with accession 18002 and 18003 recording the lowest pod numbers (4.5). Among the infected plants, accession Chinese recorded the highest pod number. All control (healthy) plants in this block had higher pod numbers than their respective infected accessions with 18001 recording the highest pod number (13.0) while Chinese had the lowest pod number of 7.5. Within the moderate watering regime, significant differences were observed on the number of harvested pods (P=0.015, F=4.38). Among the infected plants within this block, the lowest number of pods were harvested from 18001 and 18003 (3.7) and the highest from both 18002 and Chinese (5.5). Control (healthy) plants within this block recorded higher pod numbers than the infected plants with the highest (11.0) and least (6) pod numbers recorded in Chinese and 18001 respectively (Figure 7).
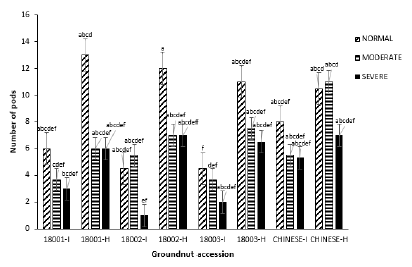
Figure 7: Mean number of pods of four groundnut accessions under the three watering regimes.
In the severe treatment block, there were no significant differences on pod numbers (P>0.05, F=2.41) with highest (5.3) and lowest (1.0) pod numbers corresponding to Chinese and accession 18002 respectively. Chinese and 18001 recorded the highest (7.0) and least (6.0) pod numbers respectively within the control (healthy) plants in this block (Figure 7). Generally, pooled mean values for number of pods across watering regimes were significant (P=0.00, F=15.95).
Seed weight: Within the normal watering regime, mean seed weight recorded for each accession were significantly different (P=0.00, F=9.55). Infected plants within this block recorded the highest (0.44 g) and lowest (0.35 g) seed weight from 18001 and 18002 respectively. The moderate treatment block also had mean seed weight varying significantly (P=0.00, F=19.34).
The highest seed weight (0.44 g) recorded within infected plants in this block was found in accession 18002 and the lowest (0.32 g) from 18003. Mean seed weight within the severe block were significant (P=0.00, F=8.29). Accession 18001 and 18003 recorded the highest (0.33 g) and lowest seed weight (0.29 g) respectively in (Figure 10). Generally, seed weight of virus infected plants in each water treatment block was lower compared to their relative control healthy plants (Figure 8).
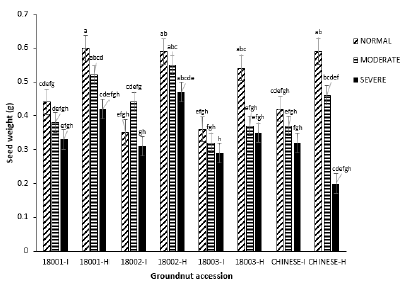
Figure 8: Mean seed weight of four groundnut accessions under the three watering regimes.
Root to shoot ratio: The mean root to mean shoot ratio of the groundnut accessions was significantly different under the three watering regimes (P=0.00, F=106.76). Infected plants of accession 18003, within the normal block, gave the least root to shoot ratio (0.28 ± 0.002) whereas all other three accessions recorded equal values of 0.03 ± 0.295. In the moderate block, accession 18001 recorded the highest (0.43 ± 0.01) whereas 18002 gave the lowest shoot to root ratio (0.38 ± 0.01). In the severe block accessions 18002 and 18001 again recorded the lowest (0.56 ± 0.05) and highest (0.82 ± 0.1) root to shoot ratio respectively (Figure 9).
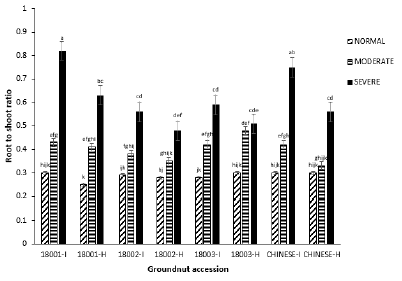
Figure 9: Mean root to shoot ratio of four groundnut accessions under the three watering regimes.
Infected roots of accessions in the normal watering appeared shorter compared to their respective counterparts in the moderate and severe watering regimes respectively (Figure 10 A-C). Similar trend was observed in roots of healthy plants in the normal, moderate and severe watering regimes (Figure 10 D-F).
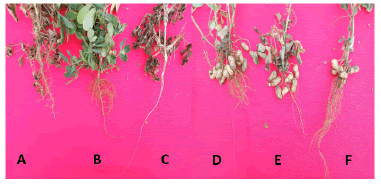
Figure 10: Differences in root length of groundnut plants under three watering regimes.
A-C are roots of infected plants under normal, moderate and severe watering regimes respectively. D-F are roots of healthy plants under normal, moderate and severe watering regimes respectively.
Discussion
Effect of virus and drought stress on disease incidence and severity
In this report, the phenomenon of increasing disease incidence and severity were observed with increasing drought stress levels which were consistent with earlier works where drought stress had been reported to cause increasing disease incidence and severity levels [13]. The relatively high disease incidence on accession 18002 in the severe block and its comparatively low incidence levels for normal and moderate blocks revealed that high disease incidence depended on the severity of water stress factor [14].
Symptoms observed during this experiment included chlorosis, reduced leaf internodes, downward curling and puckered leaflet, reduced leaf sizes and yield loss were consistent indicators of the presence of the GRV and its satellite RNA virus [5,6]. Chlorotic and dark greening of the groundnut leaflets in this work revealed that two forms of the GRD, namely chlorotic rosette and green rosette, may have been successfully grafted onto the groundnut plants.
It was also shown that with increasing number of days after planting, a concomitant increase in symptom severity scores could be observed in this research as reported in the work of Ihejirika (2007) [15]. Increase in symptom severity may further be explained by a reduced ability of plant’s defence mechanisms due to advance growth development, including flowering and seed-production, which impose a high demand for photo-assimilates and nutrients and therefore interfere with the plant’s capacity to prevent the rapid development of disease as reported by Ihejirika, (2007) [15]. This implied that the groundnut plants became more susceptible with age as they moved from the vegetative phase to the reproductive phase.
Effect of virus and drought stress on plant height and leaf area
Virus infection is known to cause severe stunting in plants and a reduction in leaf sizes [5] and drought stress is also known to cause in stunted growth in plants [16]. The action of the combined viral and drought stress factors may have brought about severe stunting and reduced leaf sizes. It is possible that drought stress may have caused damage to plants resulting in leakage of cellular nutrients into the apoplast, facilitating successful pathogen infection as reported by Ramegowda and Senthil-Kumar (2014) [17]. Moreover, plants could become vulnerable to pathogenic infection as a result of drought stress leading to total reduced surface area, shoot length and leaf water potential as compared to pathogen-alone infected plants grown under normal soil moisture [18,19].
Effect of virus and drought stress on RWC and SMC
A plant’s ability to withstand drought may be seen in its potential to retain water in its system. Relative Water Content (RWC) helps in screening for plant species resistant or tolerant to drought stress [20]. In this study, almost all accessions had lower RWC values with increasing drought stress levels and this corresponded to earlier reports by Painawadee et al, [21] who reported that plants under water deficit conditions had lower RWC. Accession Chinese recorded RWC values that did not differ greatly across all three watering regime blocks implying that it could be mildly tolerant to drought stress. Soil moisture values increased in relation to RWC values indicating that the plants which recorded higher RWC values had higher amount of moisture at their disposal for absorption by the roots as reported by Kambiranda (2011) [16].
Effect of virus and drought stress on yield
Factors such as drought, pathogen infection and hyper-salinity are known to have pronounced negative effects on plant yield [22]. Drought in groundnuts has been known to cause yield reduction due to the impediment of the formation of pegs, the organ responsible for pod production [16]. Girdthai et al. and Oteng-Yeboah et al. further stated that drought in groundnuts cause low yield when stress conditions occur prior to, or during peg formation [23,24]. Similarly, GRD is known to cause low yield in groundnut plants [25]. Pod number and seed weight recorded in this study were consistent with these findings.
Effect of virus and drought stresses on root to shoot ratio
Root to shoot ratio is a measure of the distribution of photosynthetic products into aboveground biomass and belowground biomass [26]. Under drought stress, plants have been reported to have higher root to shoot ratios as their root systems elongate and shoot systems are reduced [27]. This is to ensure that plants are able to reach deeper layers of the soil to access water [28]. Generally, this work recorded root to shoot ratio values that were lower in the normal block as a result of the small length of their root systems whereas root to shoot ratio values increased with increasing drought stress levels. This is in line with the findings of Thangthong et al [29,30]. who reported that groundnut plants under drought stress developed longer root lengths as drought stress levels increased. Accessions 18001 and Chinese recorded the highest root to shoot ratio in the severe block where drought stress was at its peak, implying that they may be better adapted to severe drought stress as compared to other accessions. Accessions 18001 and Chinese may be putatively drought resistant because they recorded the highest root to shoot ratio values with their roots reaching deeper layers of the soil in order to access any available water in the oil [31-34].
Conclusion
We concluded that response of plants to a combination of drought and pathogen was strongly varietal dependant and intensity of the drought stress. GRD symptoms including chlorosis, plant stunting, reduced leaf sizes and low yield were observed in all groundnut accessions used in this study implying that GRD agents were successfully transmitted to grafted plants. High disease incidence levels recorded were as a result of the combined effect of drought stress on virus infection, an indication of the synergistic interaction between virus and drought stresses.
Acknowledgement
Logistics support was provided by department of plant and environmental biology, school of biological sciences, University of Ghana-Legon and Biotechnology and Nuclear Agriculture Research Institute (BNARI), Ghana atomic energy commission, Kwabenya- Accra.
Declaration of Conflict of Interest
Authors declare no conflict
References
- Arya SS, Salve AR, Chauhan S (2015) Peanuts as functional food: A review. J Food Sci Technol 53: 31-41.
[Crossref] [Google scholar] [Indexed]
- Asante M, Ahiabor BDK, Atakora WK (2020) Growth, nodulation, and yield responses of groundnut (Arachis hypogaea L.) as influenced by combined application of rhizobium inoculant and phosphorus in the Guinea Savanna zone of Ghana. Int J Agron 202.
- Appiah AS, Tegg RS, Offei, SK, Wilson CR (2016a) Impact of groundnut rosette disease on nutritive value and elemental composition of four varieties of peanut (Arachis hypogaea). Ann Appl Biol 168: 400- 408.
- Okello DK, Akello LB, Tukamuhabwa P, Odong TL, Ochwo-Ssemakula M, et al. (2014) Groundnut rosette disease symptoms, types, distribution and management of the disease in Uganda. Afr J Plant Sci 8: 153-163.
- Appiah AS, Tegg RS, Offei SK, Wilson CR (2016b) Varietal response to groundnut rosette and the first report of Groundnut ringspot virus in Ghana. Plant Dis 100: 946-952.
- Mukoye B, Mabele AS (2019) Genetic diversity of groundnut rosette disease causal agents towards its management: A Review. International Journal of Genetics and Genomics 7:12-17.
- Ben-Ari G, Lavi U (2012) Marker-assisted selection in plant breeding. Plant Biotechnology and Agriculture 163- 184.
- Teshome DT, Zharare GE, Naidoo S (2020) The threat of the combined effect of biotic and abiotic stress factors in forestry under a changing climate. Front Plant Sci 11: 1874.
- Bostock R, Pye M, Roubtsova T (2014) Predisposition in plant disease: Exploiting the nexus in abiotic and biotic stress perception and response. Annu Rev Phytopathology 52: 517–549.
- Chojak-KoĆșniewska J, KuĆșniak E, Zimny J (2018) The effects of combined abiotic and pathogen stress in plants: Insights from salinity and Pseudomonas syringae pv lachrymans interaction in cucumber. Front Plant Sci 9: 1691.
- Kissoudis C, van de Wiel C, Visser RGF, van der Linden G (2014) Enhancing crop resilience to combined abiotic and biotic stress through the dissection of physiological and molecular crosstalk. Front Plant Sci 5:207.
- Owusu-Adjei E, Baah-Mintah R, Salifu B (2017) Analysis of the groundnut value chain in Ghana. World J Agric Res 5:177-188.
- Hossain M, Veneklaas EJ, Hardy GESJ, Poot P (2019) Tree host-pathogen interactions as influenced by drought timing: Linking physiological performance, biochemical defence and disease severity. Tree Physiol 39: 6-18.
- Achuo EA, Prinsen E, Höfte M (2006) Influence of drought, salt stress and abscisic acid on the resistance of tomato to Botrytis cinerea and Oidium neolycopersici. Plant Pathol 55:178–86.
- Ihejirika GO (2007) Effects of maize intercropping on incidence and severity of leafspot disease (cercospora arachidicola hori) and nodulation of groundnut. Int J Agric Res 2: 504-507.
- Kambiranda D, Vasanthaiah M, Ananga HKN, Basha RKA, Naik K (2011) Impact of drought stress on peanut (Arachis hypogaea L.) productivity and food safety. In H. Vasanthaiah (Ed). Plants and Environment.
- Ramegowda V, Senthil-Kumar M (2014) The interactive effects of simultaneous biotic and abiotic stresses on plants: Mechanistic understanding from drought and pathogen combination. J Plant Physiol 176: 47–54.
- Ghanbary E, Fathizadeh O, Pazhouhan I, Zarafshar M, Tabari M, et al. (2021) Drought and pathogen effects on survival, leaf physiology, oxidative damage, and defense in two Middle Eastern oak species. Forests 12: 247.
- Zarattini M, Farjad M, Launay A, Cannella D, Soulié MC, et al. (2021) Every cloud has a silver lining: how abiotic stresses affect gene expression in plant-pathogen interactions. J Exp Bot 72: 1020–1033.
- Zegaoui Z, Planchais S, Cabassa C, Djebbar R, Belbachir OA, et al. (2017) Variation in relative water content, proline accumulation and stress gene expression in two cowpea landraces under drought. J Plant Physiol 218: 26-34.
- Painawadee M, Jogloy S, Kesmala T, Chutipong A, Patanothai A (2009) Identification of traits related to drought resistance in peanut (Arachis hypogaea L.). Asian J Plant Sci 8: 120-128.
- Jaleel CA, Praramasivam M, Wahid A, Farooq M, Juburi HJ, et al. (2009) Drought stress in plants: A review on morphological characteristics and pigments composition. Int J Agric Biol 11: 100-105.
- Girdthai T, Jogloy S, Akkasaeng C, Vorasoot N, Wongkaew S, et al. (2010) Heritability of, and genotypic correlations between, aflatoxin traits and physiological traits for drought tolerance under end of season drought in peanut (Arachis hypogaea L.). Field Crops Res 118: 169â??176.
- Oteng-Frimpong R, Kassim YB, Danful R, Akromah R, Wireko-Kena A, et al. (2019) Modeling groundnut (Arachis hypogaea L.) performance under drought conditions. J Crop Improv 33: 125-144.
- Okello DK, Biruma M, Deom CM (2010) Overview of groundnut research in uganda: Past, present and future. Afr J Biotechnol 9: 6448-6459.
- Yulin Q, Wei W, Cungen C, Liding C (2019) Plant root-shoot biomass allocation over diverse biomes: A global synthesis. Glob Ecol Conserv 18:e00606.
- Zhang N, Zhao B, Zhang HJ, Weeda S, Yang C, et al. (2013) Melatonin promotes water-stress tolerance, lateral root formation, and seed germination in cucumber (Cucumis sativus L.). J Pineal Res 54: 15-23.
- Fang Y, Du Y, Wang J, Wu A, Qiao S, et al (2017) Moderate drought stress affected root growth and grain yield in old, modern and newly released cultivars of winter wheat. Front Plant Sci 8: 672.
- Das IK, Rakshit S (2016) Millets, their importance, and production constraints. In IK Das and PG Padmaja (Eds.). Biotic Stress Resistance in Millets pp: 3-19.
- Khayatnezhad M, Gholamin R (2011) Effects of salt stress levels on five maize (Zea mays L.) cultivars at germination stage. Afr J Biotechnol 10: 12909-12915.
- Li Y, Li H, Li Y, Zhang S (2017) Improving water-use efficiency by decreasing stomatal conductance and transpiration rate to maintain higher ear photosynthetic rate in drought-resistant wheat. The Crop Journal 5: 231-239.
- Ramegowda V, Senthil-Kumar M (2015) The interactive effects of simultaneous biotic and abiotic stresses on plants: Mechanistic understanding from drought and pathogen combination. J Plant Physiol 176: 47–54.
- Rejeb B, Pastor V, Mauch-Mani B (2014) Plant responses to simultaneous biotic and abiotic stress: Molecular mechanisms. Plants (Basel) 3: 458-475.
- Thankgthong N, Jogloy S, Jongrungklang N, Kvein CK, Pensuk V, et al. (2017) Root distribution patterns of peanut genotypes with different drought resistance levels under earlyâ?season drought stress. J Agron Crop Sci 204: 112-12.