Research Article, J Electr Eng Electr Techno Vol: 11 Issue: 7
A Low-Cost Photodiode Based Solar Radiation Measuring System
Zvinodashe TT Feresu*, Emmanuel Mashonjowa and Electdom Matandirotya
Department of Space Science and Applied Physics, University of Zimbabwe, P.O. Box MP167, Mt Pleasant, Harare, Zimbabwe.
*Corresponding Author: Zvinodashe TT Feresu , Department of Space Science and Applied Physics, University of Zimbabwe, P.O. Box MP167, Mt Pleasant, Harare, Zimbabwe; E-mail: zferesu@gmail.com
Received date: 30 December, 2022, Manuscript No. JEEET-22-56467;
Editor assigned date: 02 January, 2023, PreQC No. JEEET-22-56467 (PQ);
Reviewed date: 17 January, 2023, QC No. JEEET-22-56467;
Revised date: 25 January, 2023, Manuscript No. JEEET-22-56467 (R);
Published date: 02 February, 2023 DOI:10.4172/2325-9833.1000907.
Citation: Feresu ZTT, Mashonjowa E, Matandirotya E (2023) A Low-Cost Photodiode Based Solar Radiation Measuring System. J Electr Eng Electr Technol 12:1.
Abstract
Accurate measurements of solar irradiance are necessary for the successful design and implementation of solar energydependent systems such as solar power systems. This study presents a design of a low cost photodiode based pyranometer using commercially off the shelf components for the measurement of solar irradiance in the visible spectral range (approx. 400 to 750 nm). The design aimed to fabricate and test a low-cost pyranometer with the following principal characteristics; high accuracy, ease of connection, immunity to noise, remote programming and operation, interior temperature regulation, cosine error minimization, and portable. The designed photodiode based pyrometer was calibrated in a standard laboratory. The calibration and test results of the low cost photodiodebased pyranometer have characteristics such as sensitivity, low cost and easily controllable. The characteristics of the designed pyrometer are similar to those of thermopile based pyranometer and, therefore, can be used in any application area where reliable measurement of solar irradiance is necessary.
Keywords: Pyranometer; Photodiode; Spectral response; Calibration
Introduction
Radiation is important as a means of energy transfer for all processes. The total solar energy radiated to the Earth’s surface is important in many application areas where the processes rely on the amount of solar radiation. Such application areas include renewable energy systems as well as agricultural meteorology. Farmers, industrial processing and agricultural research groups, and many other processes need environmental data to augment their knowledge of environmental components for an area under consideration [1].
In most cases where the knowledge of solar energy radiation is needed, accurate measurement of the total of solar (mainly short-wave) and terrestrial (mainly long-wave) radiation is critical. Terrestrial radiation is emitted by plants and any other surface on earth after converting the radiation from the sun [2]. For example, in the agricultural meteorology sector, the fraction of the solar radiation which can initiate photosynthesis must be known to predict or evaluate plant growth. The component of solar radiation used in photosynthesis falls between 400-700 hm and is referred to as the Photosynthetically Active Region (PAR). The proportion of PAR in total (direct+diffuse) radiation is about 50%.
Most instruments used for measuring solar and long wave radiation consist of different forms of thermopile arrangement. A thermopile consists of a series of alternate junctions between two dissimilar metals e.g. copper and constantan. When a temperature difference exists between two sets of metals, a voltage is generated which is proportional to the temperature difference. Most radiation sensors give an output of a few millivolts and may be connected directly to recorders, data loggers or integrators. The important characteristics of radiation sensors of common concern when evaluating the accuracy and quality of radiation measurements are:
- The sensitivity
- Stability
- Response time
- Cosine effect
- Linearity
- Temperature response
- Spectral response
The use of such systems contributes to dissemination of these weather variables, recognizing them in real-time. Another way of sensing solar radiation is by using photodiodes or phototransistors [3]. This project designed and constructed a low cost solar radiation sensor using photodiodes or phototransistors. The instrument was designed and developed under this consideration of keeping the cost as low as possible to make it easily available. The photodiode-based pyranometer is to replace the very expensive commercial CM11 pyranometer in the weather station [4].
To ensure the low cost of the Data Acquisition System, (DAS) and to promote the dissemination of this technology in developing countries, the proposed data acquisition system and the software Linux and Windows operating systems are to be cautiously implemented. This includes a BPW34 photodiode for measuring solar radiation [5]. The current study focused on the design of a photodiode based pyranometer to be included in an automatic Weather Data Acquisition and Reporting System (WDARS). The proposed WDARS contains many weather parameter acquisition sensors, also designed at low cost. The parameters are sent into a microcontroller in an Arduino Uno board for processing and saving, and then sent to interested parties through the internet to be received on the users’ mobile phones and computers. The main objective is to be able to replicate and deploy these WDARS in many stations countrywide for parallel weather information processing. Such weather-related information would be necessary for the Agricultural Sector as well as for improving weather prediction and forecasting countrywide.
Materials and Methods
This design is based on photodiodes which are used to detect solar radiation. The basic principle is that these devices are very sensitive, low cost, and easily controllable through electronic circuitry. The design attempts to match the sensor characteristics to those of the Kipp and Zonen (CM11) design which is accepted internationally as basic industry standards for day-to-day monitoring of solar radiation. However, the response time of the semiconductor detectors will be ultra-short compared to that of thermopile devices. Photodiodes are manufactured with central spectral responses ranging from 300 nm to 1000 nm. Figure 1 shows the responses of the detector. The most common central wavelengths are 550 nm and 900 nm approximately. In most cases, the spectral response ranges from -50% to +100% of the central response. The solar radiation sensor consists of one such component and its output signals achieve the full range of 300 nm to 2800 nm which is covered by the CM11. The outputs of the high speed silicon made BPW34 photodiode were cautiously implemented. These were adjusted till the total response matched, as closely as possible, the spectral range and flat spectral profile of the CM11 [6].
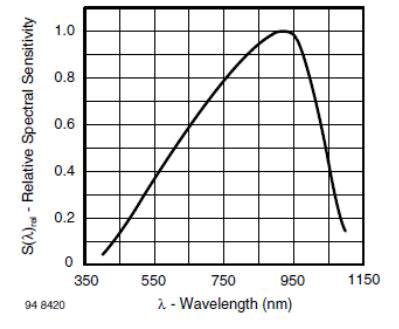
Figure 1: Relative spectral sensitivity of a photodiode.
The designed sensor output was calibrated against a commercial pyranometer (model CM11), whose calibration can be traced to a standard pyrheliometer. For the reception of the whole solar spectrum, the pyranometer and the photodiode sensor were placed on a flat roof where they were tamperproof. The outputs of the two were connected to terminals of a CR23X data logger. The inputs from pyranometer were connected so as to give radiation in Watts per square meter (Wm-2) while that from the photodiode circuit was giving results in milli-Volts (mV).
The flat response of the thermopile-type pyranometer was mimicked by carefully adjusting the sensitivity of the photodiode detector by varying the feedback resistor in the circuit (Figure 2).
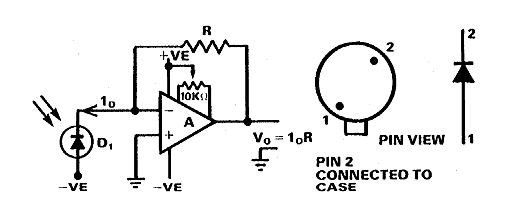
Figure 2: Product details of a photodiode.
(“[7], BPW34 IR+Visible Light Si Photodiode, 65°, Through Hole | RS Components,” n.d.)
Block diagram of the photodiode circuit
Figure 3 shows the design circuit of the photodiode sensor system. It is made up of 5 functional blocks as listed below:
Block 1: Sensing element -A Photodiode is for detecting solar radiation. It is a semiconductor p-n junction device that converts light into an electrical current
Block 2: Signal processor-This is made up mainly of an amplifier with a feedback control circuit. It is for amplifying signals from the photodiode.
Block 3: Data acquisition-The Arduino Uno board which has a microcontroller is for data acquisition and processing.
Block 4: Display-16 × 2-character Liquid Crystal Display (LCD) for displaying the processed information from the Arduino Uno.
Block 5: PSU–DC Power Supply Unit. Carbon zinc, alkaline batteries, 9V, and coin cell are types of primary batteries to be utilized in a circuit.
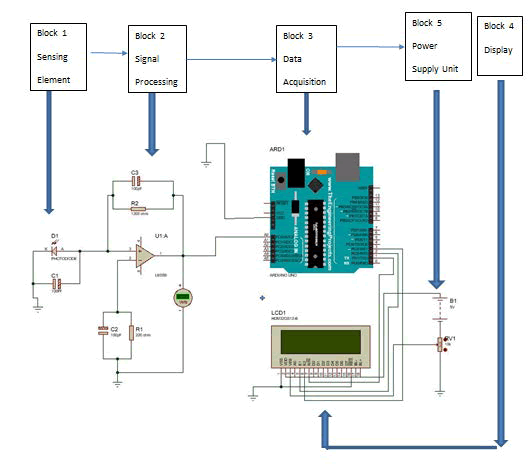
Figure 3: Circuit diagram for the solar radiation sensor.
Results and Discussion
Solar radiation sensor calibration
Calibration was achieved by plotting a graph of the photodiode results in millivolts against those of the CM11 pyranometer in watts per square meter as shown in Figure 4 below. To get the multiplier, the output of the test sensor was plotted against that of the pyranometer. The gradient (or slope) of such a graph was taken as the calibration constant for the sensor. The coefficient of determination was found close to 1 which would be perfect. The graph gives a linear relationship between the photodiode outputs in millivolts as related to solar radiation in Watts per square meter [8].

Figure 4: Diurnal variation of solar radiation as measured by the two sensors.
Validation of the results
After calibration of the photodiode sensor, the formula given from the calibration, y=1.761 × -27.05 is then used to validate the process. In validation, the multiplier and constant of the first graph were applied to the values of figures from an independent period. In validation, the values of the test component are multiplied by the equation of the first graph. This then gives a linear graph of the values of the photodiode compared to those of the CM11 pyranometer.
The days taken into consideration was the 18th of September when it was sunny throughout the day, then on the 20th of the same month when it was partially cloudy and also on the 21st of the same months when it was overcast for the whole day (Figure 5).
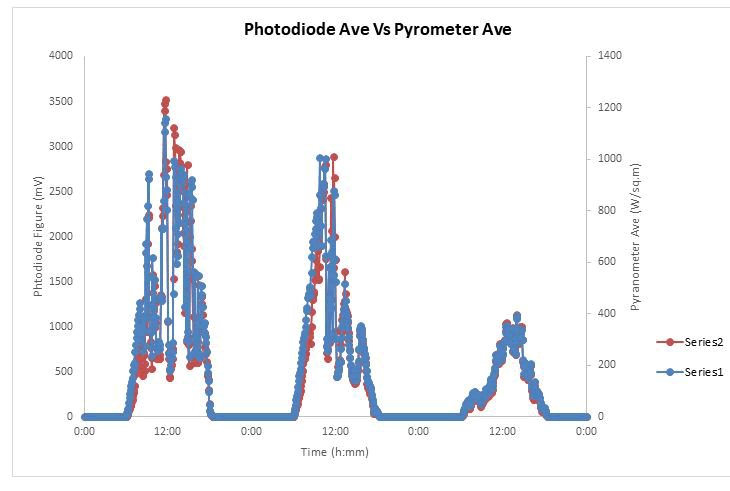
Figure 5: Validation graph of calibrated photodiode values versus those of the pyranometer. Series 1: Series of photodiode values; Series 2: Series of pyranometer values.
The graph produced was satisfactory so the photodiode was then connected to the Arduino board.
Conclusion
As indicated in Figure 4, R2 the coefficient of determination in the equation is found to be R2=0.948 which is close to unity. Figure 5 indicates how the values of the experimental pyranometer compare with those of the standard CM11 pyranometer. The validation graph shows that at low values of illumination, the values are all most equal. At high values of solar radiation, the differences are narrow and are probably due to unevenness in leveling of the physical mounting of the sensor.
References
- Duchon CE, O’Malley MS (1998) Estimating cloud type from pyranometer observations. J Appl Meteorol 38:132-141.
- Vivar M, Fuentes M, Norton M, Makrides G, De Bustamante I (2014) Estimation of sunshine duration from the global irradiance measured by a photovoltaic silicon solar cell. Renew Sustain Energy Rev 36 :26-33.
- Medugu DW, Burari FW, Abdulazeez AA (2010) Construction of a reliable model pyranometer for irradiance measurements. Afr J Biotechnol 9:1719-1725.
- Hidalgo F, Martinez RF, Vidal E, (2014) Design of a Low-Cost Sensor for Solar Irradiance.
- Patil A, Haria K, Paste P (2013) Photodiode based Pyranometer. Int J Adv Sci Eng Technol 1:29-33.
- Saito T (2011) Spectral Properties of Semiconductor Photodiodes. In: Betta, GFD, editor. Advances in Photodiodes. London: Intech Open; 2011.p.480
- Bayrakçı HC, Demircan C, Kecebas A (2018) The development of empirical models for Estimating global solar radiation on the horizontal surface: A case study. Renew Sust Energ Reviews 81:2771-2782.
- Driesse A, Zaaiman W, Riley D, Taylor N, Stein JS (2016) Investigation of pyranometer and photodiode calibration under different conditions. IEEE Conference on 43rd Photovoltaic Specialists, Portland, 0127-0132.