Review Article, J Electr Eng Electron Technol Vol: 11 Issue: 8
Economic Impact of Millimeter Wave Wireless Communication: A Review
Arebu Dejen* and Murad Ridwan
Department of Electrical and Computer Engineering, Addis Ababa University, Addis Ababa, Ethiopia
*Corresponding Author: Arebu Dejen
Department of Electrical and Computer Engineering,
Addis Ababa University,
Addis Ababa,
Ethiopia,
Tel: +251911584277;
E-mail: arbdjn@gmail.com
Received date: 30 December, 2022, Manuscript No. JEEET-22-63305;
Editor assigned date: 02 January, 2023, PreQC No. JEEET-22-63305 (PQ);
Reviewed date: 17 January, 2023, QC No. JEEET-22-63305;
Revised date: 25 January, 2023, Manuscript No. JEEET-22-63305 (R);
Published date: 02 February, 2023, DOI: 10.4172/2325-9833.1000922
Citation: Dejen A, Ridwan M (2023) Economic Impact of Millimeter Wave Wireless Communication: A review. J Electr Eng Electron Technol 12:1.
Abstract
The demand for high data rate and exponential growth of mobile data trafficking, which creates scarcities of bandwidth under microwave frequency experienced by wireless communication has motivated to invoke millimeter-wave (mm- Wave) spectrum for future 5G broadband mobile communication networks. This survey paper review the research works on the characteristics of the mm-Waves propagation channel and highlights the main challenges, solutions, and benefits associated with the use of mm-Waves, and their performance analysis. The paper also discusses proper technical implementation strategy and economic benefits from the operator side.
Keywords: 5G; mm-wave; Use case and scenario; Economic
analysis
Introduction
The evolutions of wireless data applications and the increasing popularity of smart devices have led to a massive proliferation in mobile data traffic (Figure 1), creating radical challenges for mobile service providers [1]. With the advent of the Internet of Things (IoT), it is foreseen that mobile traffic will witness 1000 fold increase by the year 2020 [2]. This spectacular rise calls for drastic improvement in mobile network capacity beyond the current 3G/4G networks to the next generation of wireless radio standards.
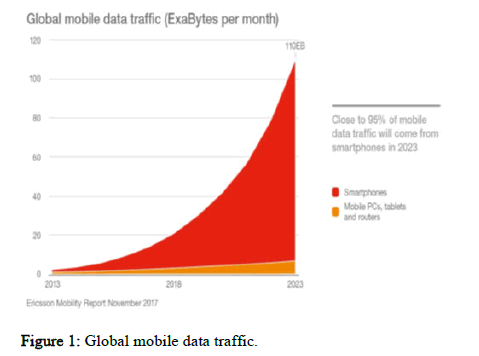
Figure 1: Global mobile data traffic.
The forthcoming 5th generation (5G) is considered an integrative system that combines the already deployed wireless communication systems under a brand new air interface. New network requirements are expected to rise due to the massive growth expected in connected devices as well as the substantial increment in the traffic volume anticipated shortly. Based on the fundamental technical challenges derived from user related concerns, end user key performance indicators are utilized to suggest candidate solutions that assess radio link requirements. These are characterized by several specifications including traffic volume density, latency, reliability, experienced enduser throughput, and availability.
New systems that cleverly use the evolved technologies and combine novel techniques are the ones that form the foundations for 5G. Specifically, the three most significant candidate technologies which boost the performance of the 5G cellular networks are the extensively increasing bandwidth. This cellular network demand will be achieved by mm-wave spectrum bands.
The mm-wave band (30 to 300 GHz) offers a vast amount of spectrum out of which an approximate 100 GHz spectrum is available for mobile communication use. This available spectrum enhances channel capacity. Whereas most of the existing mobile communication systems nowadays are deployed in the sub-6GHz spectrum which is known to be heavily crowded. To provide the required data rates and throughput and to avoid congestion, the attention of radio system designers has moved toward higher frequencies.
The other benefit of mm-wave technology is that a large number of antenna elements can be packed into a small physical size leading to a larger aperture and achieving high antenna array gains. Coupled with Massive MIMO and femtocells, mm-Waves can be the key solution for future 5G mobile communication. Nevertheless, some issues and questions must be addressed for such a system to become a reality [3].
In principle, the data rate is depending on available Bandwidth for transmission (BW) and Signal to Noise Ratio (SNR) as the Shannon equation sates.
Increasing bandwidth is an obvious way to improve the channel capacity in common. However, it also increases the noise in the channel and therefore reduces the signal to noise ratio at a fixed signal level.
Where pt transmitted power, Gr receiver gain, Gt is transmitter antenna gain, d separation distance, α channel factor, λ propagation wavelength, K Boltzmann constant and is equal to 1.38 ×10−23J/K, T is the absolute temperature. NF is the noise factor of the receiver.
Due to high penetration and path loss, mm-wave signals oblige to work at a short distance. This is advantageous for frequency reuse, and better security and privacy.
Implementation Challenges
Even though the mm-wave band, seems to be an auspicious candidate for the next-generation 5G cellular system, which is expected to support high data rates of multiple Gb/s. However, employing mm-Wave requires dealing with the propagation attributes and the channel impairments of the high frequency bands. Major impediments of mm-wave propagation are higher path loss due to higher carrier frequency; reduced scattering which in turn reduces the available diversity, and increased effect of blockage as a result of weaker non-line of sight paths. In addition, the effect of noise power is more pronounced due to the usage of larger bandwidths.
Propagation loss
Free Space Path Loss (FSPL): The free space path loss is dependent on the carrier frequency fc. Increasing the carrier frequency will reduce the antenna size. As a result, the effective aperture of the antenna scales by a factor of λ2/4π, while the free space path loss grows with fc2. Hence, increasing the carrier frequency fc from 3 to 30 GHz, will correspondingly add a power loss of 20 dB regardless of the transmitter-receiver distance.
Increasing antenna aperture, by building an electrically large antenna/antenna array provides sufficient antenna gain and directivity in both [BS and UE]. This will overcome the path loss and ensure high SNR output. Since free space path loss is inversely proportional to antenna aperture. Massive MIMO and Beam-forming are possible for the mm-wave antenna.
Blockage: Microwave signals are less vulnerable to blockages but they fade due to diffraction. In contrast, mm-Wave exposes prismatic propagation and suffers less diffraction than the microwave signals, making them much more susceptible to blockages.
• They suffer much higher penetration losses
• Do not diffract well in terrestrial environments
• Required directionality, both the transmitter and receiver beam patterns are focused over a more narrow beam width
Atmospheric and rain absorption: A major impediment to mm- Wave communications is attenuation due to rain, foliage, and atmospheric absorption. Atmospheric attenuation due to oxygen absorption or heavy rain can be on the order of 10-20 dB/km (Figures 2 and 3) [4,5].
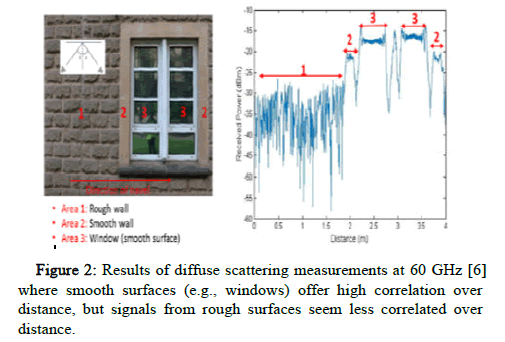
Figure 2: Results of diffuse scattering measurements at 60 GHz [6] where smooth surfaces (e.g., windows) offer high correlation over distance, but signals from rough surfaces seem less correlated over distance.
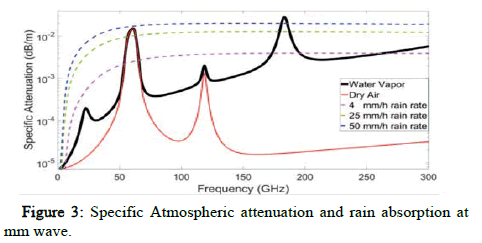
Figure 3: Specific Atmospheric attenuation and rain absorption at mm wave.
To overcome blockages, and coupled with the use of adaptive steerable arrays, mm-wave networks can also be made very dense and can benefit from the current trend of moving the cellular system to a more heterogeneous infrastructure that includes small cells and relays. Small size cells known as femto and picocells allow higher frequency reuse rates with a range of 10-200 m. The atmospheric absorption nature of the mm-wave will efficiently increase the isolation of each cell by further attenuating the background interference from more distant base stations.
Interference and its management
Intra-Cell Interference: It is a challenge between User Equipment (UEs) with other User Equipment (UEs). It can be mitigated by proper scheduling and beam forming design.
Inter-Cell Interference: At the cell edge adjacent cells causes strong interference between cells in mm-wave cellular networks, scheduling based on time frequency space resource with fully directional communication reduce it. On demand interference management strategy (UEs and BTs).
Inter-Layer Interference: It refers to interference among different layers, macro, micro, femto, and pico cells. This demands careful design of the pilots and control messages to avoid inefficient utilization of the available resources.
Spectrum Aspects: Beyond the traditional sweet spot of spectrum for wireless communications which ends at around 6 GHz, there is an additional 100 GHz of spectrum in the so-called mm-wave frequency range that today is mainly under-utilized. This spectrum band has channel sizes capable of supporting wireless data speeds of 10-50 Gbps [7].
A significant proportion of this enormous radio spectrum could be unlocked by 5G cellular and WiFi communications. For the field of 5G research, the target is above 6 GHz, and the research in this field should look to Electromagnetic Field (EMF) aspects, link budgets, propagation issues, and channel model description. In the following, a low millimeter band and high millimeter bands within the range of 20 to 90 GHz are briefly discussed.
At 28 GHz there is available 1.3 GHz. And at 39 GHz about 2.1 GHz spectrums are accessible. As you see in Figure 4. there is also a 7 GHz unlicensed spectrum surrounding 60 GHz. Additionally, ITU has allocated lightly licensed 5GHz bandwidths for both 71-76 GHz and 81-86 GHz bands for establishing high bandwidth wireless links, [9-11]. Similarly, the band 92-95 GHz has been allocated for high bandwidth wireless links .
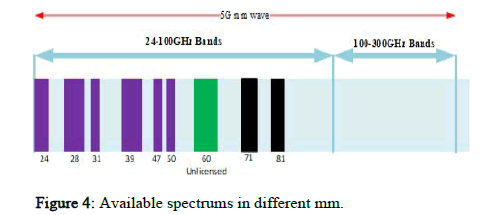
Figure 4: Available spectrums in different mm.
Although mm-wave technologies at 28 GHz and 60 GHz have the potential to be used for early deployment of 5G in South Korea, the US, and Japan, there is a need for deeper discussions regarding the scenarios, use cases, and corresponding enabling technologies.
Use case and scenario
Mm-wave could be implemented for short-latency applications and broadband applications with stationary, high, and ultra-high-speed mobility users.
Indoor coverage scenarios: The indoor scenario can be classified as an isolated room and a large public area. The isolated room includes an exhibition hall, production workshop, and offices. It can be providing full coverage of the space by a small number of mm-wave Nano-cells. In a large public area, the multiple numbers of mm-wave Nano cells could cover a full coverage of the area. It includes malls, shopping centers, sports facilities, airports, stations, and enterprises.
Outdoor coverage scenarios: A very large urban scenario with traditional LTE/LTE-A base stations sharing the same area with mmwaves small-cells to supply localized high rate coverage.
Dense urban scenario: It is a place users tend to gather and move as large and dynamic crowds while wanting to keep connectivity to the cloud. This is one of the important scenarios in 5G. Requires dense mm-wave access points because of the high traffic volume generated by smartphones and tablets, sensors and wirelessly connected cameras, and others. Typical environments are open squares, streets, stations, etc (Figure 5).
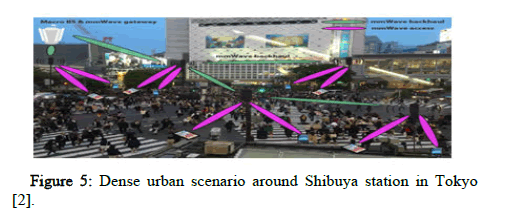
Figure 5: Dense urban scenario around Shibuya station in Tokyo [2].
Coverage probability
The coverage probability which is equivalent to P (SINR>Threshold SINR) on targeted SINR. The SINR is based on the following metrics.
Receiving antenna gain, transmitting antenna gain, the noise power, and the small scale fading are all considered in SINR calculation. The coverage probability is approximated as [12].
Due to high penetration losses and sensitivity to blockages, the coverage areas of mm-wave BSs are generally small and irregular, as shown in Figure 6. This issue can be overcome by densification of the network. When the BS density is low, BS densification decreases the distance of the serving BS and increases the probability of serving BSs being LOS BS.
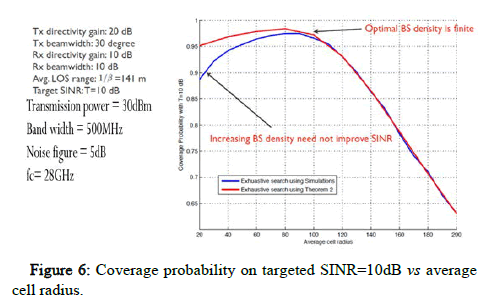
Figure 6: Coverage probability on targeted SINR=10dB vs average cell radius.
The simulated result of coverage probability mm wave with average cell radius is shown in Figure 6.
This results in higher serving power to a typical user. The interference power, however, is not affected significantly as the interferers are still far enough to be NLOS to the typical user. Therefore, densification generally helps mm-wave systems and improves their SINR and rate coverage. After a certain threshold, though there will be enough interferers in the LOS region causing the SINR to degrade significantly. The coverage probability will decrease when the average cell radius is far from the BS. Up to 200 m, there is a possibility to get a targeted SINR.
Capacity estimation
One of the basic advantages of the mm-wave is enhancing channel capacity. The following Figure 7, Show how the capacity enhancement is possible in a 28 GHz mm-wave network by just reducing antenna beam width from 65â?? to 30â?? [13]. Mm-wave 5G networks will employ multi-beam RF signals, with arbitrary beam width much less than 30â??. Therefore, it is expected that this narrowing of antenna beam width transmission strategy alone can scale up capacity significantly and help realize gigabits per second data rates everywhere in the 5G network, including cell edges [14].
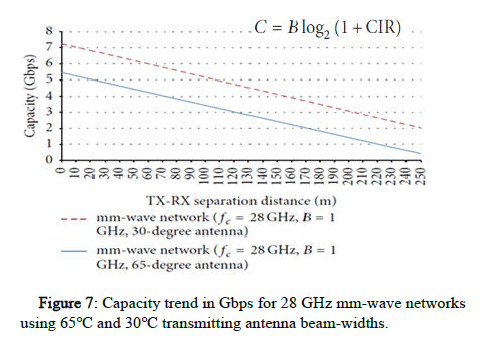
Figure 7: Capacity trend in Gbps for 28 GHz mm-wave networ ks using 65°C and 30°C transmitting antenna beam-widths.
For indoor coverage, cell enhancers and in-building RF infrastructures may be needed to ensure providing acceptable received power levels inside buildings with satisfying in-building data rates.
Technical implementation strategy
Taking care of the following preliminary works should be considered to implement mm wave technology.
Set target and deployment area: In this stage the operator should fix the target where and when used the mm wave technology. The operator may select a place of potential customers, dense populated areas and others.
Mm wave cellular network modeling: Analysis of mm-wave performance over a specific area. This will realize the draft design over all the design parameters. Most commonly used modeling Techniques.
• Stochastic geometry tool for locate cells
• Poisons Point Process (PPP) for BS location distribution
• Use random shape theory to model buildings
• Building distribution by PPP
• User equipment distribution by PPP
Network optimization: The available human resource to operate and optimize over the technology should be noted.
Market status of mm-wave
The millimeter waves or the millimeter band is quite useful in the wide range of applications such as transmitting large quantity of data over computers, cellular communication, and radar owing to its propagation characteristics as well as high frequency. Transmission of large amount of data, being one of necessity of the current time, millimeter waves are extensively used across the telecommunication sector making it one of the major applications worldwide. As there is an increasing data or information transfer being carried out nowadays, this huge information transfer requires higher frequencies. Therefore, millimeter waves come into focus due to the larger frequencies offered by them, making it a noteworthy reliable and efficient source for high frequency data transmission applications over the computers, voice channels, or televisions [15,16].
The millimeter wave technology market is segmented into five different levels, product, component, application, frequency band, and region (Figure 8).

Figure 8: Market segmentation and scope of mm wave technology.
The US mm-wave technology market will increase exponentially till 2025 as the following Figure 9 states. All the forecasting data are based on 2016 statics (Grand view research organization, USA).
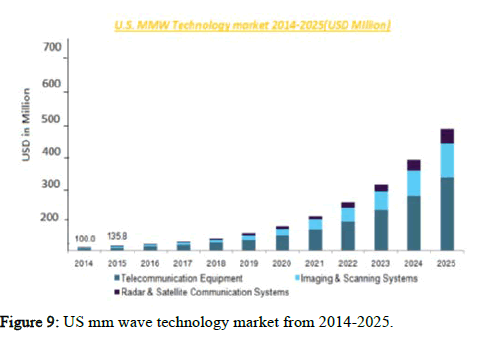
Figure 9: US mm wave technology market from 2014-2025.
Economic benefits
As far as mm-wave technology is the new 5G component, all the network structures will be updated by the new operating frequency. In addition, densification is one of the solutions for mm-wave challenges. All this will increase the capital expenditure for operators. However, to realize the customer demand for a high data rate mm-wave will be deployed once a time. So, the following capital expenditure minimization strategies will become in hand.
• The regulatory body allow spectrum sharing it helps operators to reduce their expenses by sharing the license costs.
• Industries have produced high efficient and low cost Silicon based mm wave RFIC that replaces costly GaN and GaAs
• Infrastructure sharing reduces Operational Expenditure (OPEX)
The income of the operator will increase due to the diversity of the 5G applications and related service requirements in in unexpected quality of service in terms of data rate, latency, reliability, and other parameters are fulfilled. This will lead the mm wave operator profitable since Increasing in number of subscriber and Average revenue per use for the operator.
Where ARPU→ Average Revenue per User, OPEX→ operational expenditure it includes utility services cost, overhead cost, manpower. CAPEX→ Capital Expenditure like initial investment cost for the technology including spectrum license cost.
Conclusion
Early application of mm wave communication were restricted to niche areas due to its high cost, complexity and perceived limitations. However, the need to address high data rate and low latency requirement in 5G communication has brought renewed focus to the mm wave band. Advancement in semiconductor technology and system architecture along with the development of innovative will be a key enabler as 5G emerges.
Mm-Wave cellular networks have the potential for high coverage and capacity as long as the infrastructure is densely deployed and using more sophisticated beam forming strategies. It can be deployed in different scenario to fill the gap between customers requirement whereas operators challenge, which is the demand of high data rate.
References
- Andrews JG, Buzzi S, Choi W, Hanly SV, Lozano A, et al. (2014) What will 5G be?. IEEE J Sel Areas Commun 32:1065-1082.
- Rappaport TS, Sun S, Mayzus R, Zhao H, Azar Y, et al. (2013) Millimeter wave mobile communications for 5G cellular: It will work!. IEEE access. 1:335-349.
- Rappaport TS, Xing Y, MacCartney GR, Molisch AF, Mellios E, et al. (2017) Overview of Millimeter Wave Communications for Fifth-Generation (5G) Wireless Networks-with a focus on Propagation Models. IEEE Trans Antennas Propag 65:6213-6230.
- Al-Ogaili F, Shubair RM (2016) Millimeter-Wave Mobile Communications for 5G: Challenges and Opportunities. 2016 IEEE International Symposium on Antennas and Propagation (APSURSI), 26 June 2016 - 01 July 2016 Fajardo, PR, USA.
- Gupta AK, Andrews JG, Heath RW (2016) On the feasibility of sharing spectrum licenses in mmWave cellular systems. IEEE Trans commun 64:3981-3995.
[Googlescholar] [Crossref] [Indexed]
- Rangan S, Rappaport TS, Erkip E (2014) Millimeter-wave cellular wireless networks: Potentials and challenges. Proceedings of the IEEE 102:366-385.
- Bai T, Heath RW (2014) Coverage and Rate Analysis for Millimeter Wave Cellular Networks, IEEE Trans Wirel Commun 14:1100-1114.
- Bogale TE, Le LB (2015) Massive MIMO and Millimeter Wave for 5G Wireless HetNet: Potentials and Challenges. arXiv preprint arXiv:1510.06359.
- Rebatoy M, Mezzavilla M, Rangan S, Zorziy M (2016) The Potential of Resource Sharing in 5G Millimeter-Wave Bands. arXiv preprint arXiv: 1602.07732.
- Nassar AT, Sulyman AI, Alsanie A (2015) Radio Capacity Estimation for Millimeter Wave 5G Cellular Networks Using Narrow Beamwidth Antennas at the Base Stations. Int J Antennas Propag 2015.
- Matalatala M, Deruyck M, Tanghe E, Martens L, Joseph W (2015) Performance Evaluation of 5G Millimeter-Wave Cellular Access Networks Using a Capacity-Based Network Development Tool. Mob Inf Syst 4:1-11.
[Crossref]
- Bai T, Alkhateeb A, Heath RW (2014) Coverage and capacity of millimeter-wave cellular networks. IEEE Commun Mag 52:70-77.
[Googlescholar] [Crossref] [Indexed]
- Rappaport TS, MacCartney GR, Samimi MK, Sun S (2015) Wideband millimeter-wave propagation measurements and channel models for future wireless communication system design. IEEE Trans Commun 63:3029-3056.
- Andrews JG, Bai T, Kulkarni MN, Alkhateeb A, Gupta AK, et al. (2017) Modeling and Analyzing Millimeter Wave Cellular Systems. IEEE Trans Commun 65: 403-430.
- Venugopal K, Valenti MC, Heath RW (2016) Device-to-device millimeter wave communications: Interference, coverage, rate, and finite topologies. IEEE Trans Wirel Commun 15:6175-6188.
- Vuppala S, Biswas S, Ratnarajah T (2016) An analysis on secure communication in millimeter/micro-wave hybrid networks. IEEE Trans Commun 64:3507-3519.