Research Article, J Chem Appl Chem Eng Vol: 6 Issue: 1
Hg (II) Selective Electrode Based on Poly-O-Toluidine Tin-Zirconium (IV) Molybdophosphate Nano- Composite
Rupinder Kaur, Sandeep Kaushal, Prit Pal Singh*
Department of Applied Medical Sciences, Albaha University, Albaha, Saudi Arabia.
*Corresponding Author: Prit Pal Singh
Department of Applied Medical Sciences, Albaha University, Albaha, Saudi Arabia, E-Mail: Pritpal78@gmail.com
Received date: 02 January, 2022, Manuscript No. JCACE-22-53149;
Editor assigned date: 4 January, 2022, PreQC No. JCACE-22-53149 (PQ);
Reviewed date: 11 January, 2022, QC No JCACE-22-53149;
Revised date: 18 January, 2022, Manuscript No. JCACE-22-53149 (R);
Published date: 28 January, 2022, DOI: 10.4172/jcace.1000149
Citation: Kaur R, Kaushal S, Singh PP (2021) AHg (II) Selective Electrode Based on Poly-O-Toluidine Tin-Zirconium (IV) Molybdophosphate Nano-Composite. J Chem Appl Chem Eng 5:1.
Abstract
Present work deals with the fabrication and potentiometric studies of a novel Hg (II) ion selective electrode based on nano-composite poly-o-toluidine tin-zirconium (IV) molybdophosphate. The synthesized material was characterized through the instrumental techniques viz. FT-IR, FE-SEM, TEM, XRD and TG/DTA. The higher IEC (2.40 meq/g) and selectivity for mercuric ions, prove its best candidature for the fabrication of a Hg (II) ion selective electrode. The designed electrode follows the Nernstion response over a wide concentration range (1×10‒7 M to 1×10‒1 M) and pH (2.5 to 8.0) with a slop of 24.82 mV/decade. The response time of proposed electrode was observed to be 16 seconds and it can be used effectively at least for four months without any measurable change in its potential response. The lower detection limit of electrode is 3.55×10‒8 M. The analytical importance of ISE has been established by employing it as an indicator electrode for the estimation of Hg (II) ions in the industrial waste
Keywords: Organic-inorganic composite material, PTD/SnZrMoP, Ion selective electrode, Response time; Potentiometric titrations
Introduction
Though the term ‘heavy metals’ is less precisely defined. But more often, the term is simply used to indicate metals that are toxic. Among the various heavy metals Hg proved to be the most toxic because of its rapid methylation in the aquatic environment by some bacteria. Its poisoning may lead to diseases like: Hunter-Russell syndrome, Acrodynia and Minamata, mercury poisoning may also cause a permanent damage to brain and kidney, Mercury poisoning can cause brain damage to the fetus and can lead to a condition called Erethism characterized by jerking, irritability, mental and emotional disturbance [1-4].
A large number of instrumental techniques are available for the chemical surveillance of mercuric ions in solutions [5-9]. All these detection methods are very attractive due to their higher detection limit and reasonably good selectivity for a particular ionic moiety but their limitations like, high operational cost, complicated instrumental setup and non-satisfactory results in the presence of interfering ions etc., prove them unfit for routine use. All these limitations are posing the requirement of a probe for Hg (II) ions which should be simple, reproducible and inexpensive in nature. In the reported literature a number of ion selective electrodes based on conventional membrane were used for the estimation of Hg (II) ions. It has also been observed that most of these probes depends upon the chelating (toothed ligands) agent which form the complexes with metal ions or use ionophores or neutral carriers [10-13]. The major drawback of these electrodes is their short lifetime (working) due to the loss of these chelating agents from the membrane by leaching. Now-a-day the potentiometry is considered to be the most sensitive, highly-selective, fast, eco-friendly and cost effective method for estimation of Hg2+ ions in the aqueous medium, but by making the use of such probes the estimation of Hg2+ ions at ppm range is possible but have not been proven effective for the estimation of mercury ion concentration in ppb range. In the recent years S. Kaushal [14] and A.A. Khan [15-17] reported their work on the use of organic-inorganic nano-composite ion exchangers for the fabrication of Hg2+ ion selective electrode.
In the present study, organic-inorganic nano-composite poly- o-toluidine incorporated tin-zirconium (IV) molybdophosphate was synthesized through the sol-gel technique, the powder material was used to fabricate the IEM, the membrane material was tested for various physiochemical properties by changing binder: resin, ratios and the best selected membrane material was further used to design the Hg (II) ion selective electrode. As an analytical application the proposed sensor was used for the potentiometric estimation of Hg
(II) ions titrimetrically in waste water samples collected from different industrial units. To the best of our knowledge, no such ISE based on organic-inorganic nano-composite of tetravalent metal acid salt with two anions and two cations has been reported previously for estimation of Hg (II) ions in aqueous medium.
Experimental
Reagents and instruments
The chemical reagents and solvents used in this study were of analytical reagent grade and used as received, without any further purification. Some important reagents used are, zirconium oxychloride, stannic chloride, sodium molybdate, sodium dihydrogen phosphate, o-toluidine, potassium persulphate, disodium salt of ethylene diamine tetra acetic acid (EDTA), epoxy resin, mercuric nitrate etc.
Synthesis of PTD/SnZrMoP nano-composite ion exchanger
The nano-composite poly-o-toluidine tin-zirconium (IV) molybdophosphate was prepared by the sol-gel method, by the incorporation of green gel of poly-o-toluidine [14] into the white precipitates of SnZrMoP [18] with constant stirring at room temperature (25 ± 2 ºC) [19].
Ion exchange capacity
The ion exchange capacity was determined by the standard column process by making the use of glass wool supported column. The hydrogen ions were eluted with NaNO3 solution and titrated against a standard solution of sodium hydroxide (0.1M) using phenolphthalein as indicator
Characterization techniques
The common analytical techniques viz. FT-IR, Powder XRD, FE- SEM, HR-TEM, and EDX spectroscopy were employed to study the morphology, topography, elemental composition and particle size of nano-composite ion exchanger. TG/DTA studies were performed to investigate the thermal behavior of nano-composite ion exchanger.
Distribution studies
The distribution coefficients (Kd) of various metal ions on the nano-composite were determined in different solvent systems [19] to investigate the selective nature of nano-composite ion exchanger
Fabrication of PTD/SnZrMoP nano-composite based ion exchange membrane (IEM)
Nano-composite based heterogeneous ion exchange membrane was prepared by solution casting technique. The nano-composite sample with high ion exchange capacity was used for the synthesis of IEM. The material was ground into a fine powder form and then mixed with epoxy resin. The prepared slurry was mixed thoroughly for the even mixing of nano-composite in the binding material. After even mixing the slurry was spread into two folds of parchment paper. A pressure of 2.0 kg cm-2 was applied over the glass slabs for 24 h. As the parchment membrane is non-sticky in nature and thus can be easily removed. The smooth sheet of fabricated membrane, was cut with the help of a sharp edge knife into circular discs of about 18 mm diameter. The membrane discs which have good surface qualities were selected for further investigations [14].
Physical characterization of synthesized ion exchange mem- brane (IEM)
The fabricated membranes were tested for the parameters like, membrane water content, thickness, swelling, porosity, Ion exchange capacity etc. after the conditioning of the membrane. The effect of resin ratio loaded on the physical properties of the membrane was also investigated.
Water content
The membrane was equilibrated in DMW for 24 h to elute the diffusible salts present in the membrane. After the complete removal of surface water noted down the weight of water soaked wet membrane, and in the next successive step the wet membrane was dried under vacuum condition at the temperature of 60 Ë?C until a constant weight is obtained. The weigh difference of dry and wet membrane was evaluated.
Thickness and swelling
Screw gauge is used to find out the average thickness of the membrane, whereas the swelling property of the fabricated membrane was determined by noting down the difference between the average thickness of dry membrane and membrane equilibrated in 1 M NaCl for 24 h.
Porosity
It is the property used to estimate exchange behavior and mechanical strength of fabricated membrane. Porosity (ε) is regarded as the volume of water incorporated in the cavities per unit membrane volume
Ion exchange capacity
The ion exchange capacity of heterogeneous, homemade, H+ ion saturated, ion exchange membrane was calculated through the batch process [14].
Fabrication of ion-selective electrode
All the fabricated membranes were tested for the properties like: thickness, water content, porosity, IEC, swelling, etc., and the membrane which have the best ion exchange capacity and greater physical strength was used to fabricate the ISE. The membrane was cut into a disc shape and afterward fixed at the bottom of a Pyrex glass tube (of outer diameter 1.6 cm and inner diameter 0.8 cm) with the epoxy resin, the whole assembly was allowed to dry for 24 h at room temperature. Fabricated electrode was then equilibrated with Hg(NO3)2 (0.1 M) for 7 days. The glass tube was filled 3/4 with 0.1 M Hg(NO3)2 solution. To make the electrical contact a saturated calomel electrode was inserted in the tube and another calomel electrode was inserted in the test solution.
Characterization of ion selective electrode (ISE)
The following parameters are generally evaluated to characterize an ion selective electrode:
Response time
The response time for ISE was determined by making the use of two standard solutions of Hg(NO3)2, the electrode was first inserted in the solution of molar concentration 1×10−3 M, and in next consecutive step the electrode is immediately shifted to another solution of molar concentration 5×10−3 M. The first electrode potential value was recorded at zero interval and the next at the interval of 5 seconds. The recorded electrode potential was plotted as a function of time. The time interval at which the electrode potential attains a constant value is known as the response time of the electrode [20,21].
Slope and detection limit
Potential measurements were made for the series of standard solutions of Hg(NO3)2 in the range of 1×10−10 M to 1×10−1 M, prepared by the dilution of stock solution, as described by IUPAC the commission for analytical nomenclature [22]. The recorded values of electrode potential were plotted against the log of the activities of analyte solution. The reproducibility of the system was verified by plotting the calibration curves thrice. All the measurements were performed by keeping the internal solution concentration fix at 5×10−2 M. After the completion of the process the electrode kept preserved in a 0.1 M solution of Hg(NO3)2 [14].
Effect of change in internal solution concentration
The response of proposed electrode was also investigated by changing the concentration of solution filled inside tube of ISE. The concentrations of internal solutions of Hg(NO3)2 were kept as: 1×10−2, 5×10−1 and 1×10−3 M. The value of electrode potential obtained was plotted against the log of concentration analyte solution [14].
Effect of partially non-aqueous medium
The effect of partially non-aqueous medium containing acetone or ethanol mixed with water on the response of the proposed sensor was also investigated by varying the concentration of Hg(NO3)2 from 1×10â??1 to 1×10â??10 M [14].
Effect of pH
For this study of proposed electrode a series of solutions were prepared by adjusting the pH between 1−13, by adding a few drops of 0.1 M HCl or 0.1 M NaOH solution as required, during these investigations the concentration of Hg(NO3)2 solution was kept constant in the test solution (1×10−3 M) [14].
Selectivity coefficients
Potentiometric selectivity coefficient (K_(A,B)^Pot), measure the response of the proposed electrode towards the primary ions in the presence of other interfering ions. Fixed interference method (FIM) was used to find out the value of selectivity coefficients [23]. In this process the concentration of interfering ions was maintained 1×10−4
1.According to this method, a calibration curve has to be plotted by varying the primary ion concentrations in a fixed background concentration of interfering ions. The linear calibration curve of the electrode, which is the function of the activity of the primary ions, even in the presence of other interfering ions, should be extrapolated until at the lower detection limit.
Storage and lifetime of electrode
The electrode is stored in distilled water when not in use. For the further use the electrode is first activated, by keeping it immersed in the respective metal ion solution (0.1 M) for two hours. The electrode is washed thoroughly with distilled water, before every use. The lifetime of the electrode was tested by testing the variation in its slop and response time for the interval of 15, 30, 60, 90 and 120 days. It is expected that there should not be any significant variation in the response of the proposed electrode.
Potentiometric titrations
The analytical importance of fabricated ISE can be established by using it as an indicator electrode for the potentiometric estimation of Hg (II) ions in a test solution against EDTA as titrant. The electrode potential value should be plotted against the volume of EDTA solution used [14]. The practical utilization of the ISE was also proved through its use in the potentiometric estimation of Hg (II) ions in the water samples collected from different industrial units.
Quantitative estimation of Hg (II) ions from industrial effluents
As an environmental application, the proposed electrode was also used for the potentiometric estimation of Hg (II) ions in the water samples collected from different industrial units. For this study the effluent samples were collected from, CFL manufacturing unit Ambala (India), thermometer manufacturing unit Ambala (India) and thermal power plant effluents Roop Nagar India). All the samples were first filtered out to remove all the suspended impurities and then neutralized. The color producing substances were removed by adsorption on charcoal. In the first attempt Hg (II) ions in the test samples were estimated through the simple complexometric titrations by making the use of PAN-(1-(2-Pyridylazo)-2-naphthol) indicator and a buffer solution of pH ≈ 3. Then the same titrations were performed by the use of fabricated Hg (II) ion selective electrode based on nano-composite PTD/SnZrMoP as an indicator electrode.
The experiment was repeated thrice to check the validity of proposed electrode.
Quantitative separation of Hg (II) ions from the water samples collected from different Industrial effluents on the column PTD/ SnZrMoP nano-composite ion exchanger
The effluent samples were collected from: CFL unit waste water and thermometer unit waste and thermal power plant effluents. After purification and neutralization a fixed volume of the samples collected from different industrial units was passed through the glass wool supported columns of PTD/SnZrMoP at a flow rate of 0.5 ml min−1.. The effluent were collected and recycled thrice. The metal ions were eluted with 1.0 M HNO3 solution.
Results and Discussion
Physio-chemical properties of nano-composite PTD/SnZr- MoP
The present work includes various aspects of the novel, Hg (II) ion selective electrode based on nano-composite cation exchanger poly-o-toluidine tin-zirconium (IV) molybdophosphate. The nano- composite material was synthesized through the sol-gel technique which is a less time consuming, and cost effective technique for the synthesis of nano-particles. The nano-composite was synthesized through the mixing of green organic gel of poly-o-toluidine into the precipitates of tetravalent metal acid salt SnZrMoP with two anions and two cations. The conditions of synthesis were optimized by varying the mixing volume ratios of the reactants and sample K-5 was observed to possess the maximum ion exchange capacity (2.40 meq/g) and was selected for further studies [19].
ZC – zirconium oxy-chloride; SM - sodium molybdate; SDP - sodium dihydrogen phosphate; OT - ortho- toluidine; PP - potassium persulphate; SC - stanic chloride; EDTA
– disodium salt of ethylene diamine tetra acetic acid; M - mol L−1
The higher ion exchange capacity of nano-composite PTD/ SnZrMoP might be due to the interaction between organic and inorganic polymers, which provided it an improved mechanical quality and higher number of exchangeable sites. In this synthesis the use of EDTA was made, which is acting as capping agent as well as a polymer which might also be responsible for its enhanced IEC than its organic and inorganic counterparts [24-28]. The synthesized material was also compared with other materials of same nature.
The nano-composite PTD/SnZrMoP was characterized by the instrumental technics like, XRD, EDX, FE-SEM, HR-TEM, and FT-IR spectroscopy. In the FTIR spectra of nano-composite PTD/ SnZrMoP(Figure 1), the Peaks recorded in the vicinity of, 3438, 1620, 1051, 500-650 cmâ»1 corresponds to the inorganic counterpart (SnZrMoP), whereas the peaks at 1627 and 2924 cmâ»1 wave number, correspond to organic moiety (poly-o-toluidine). The band for polar O-H group appears at ~3438 cmâ»1 and the presence of water of crystallization in the nano-composite was indicated by a peak at wave number ~1620 cm−1. A band in the region 1627 cm−1corresponds to in plane bending vibration of –NH2 group of o-toluidine, whereas the band at 2924 cm−1 attributes to the C-H stretch modes of substituted methyl group [29]. Metal-oxygen, streching vibrations were confirmed by the assembly of peaks in the region 500-650 cmâ??1 and a broadband at 1051cmâ»1may be due to the presence of POâ??3â??, HPOâ??2â??, Hâ??POâ??1â?? groups. [30,31].
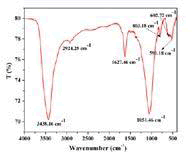
Figure 1: FTIR spectra of PTD/SnZrMoP nano-composite ion exchanger
FE-SEM images of the nano-composite material PTD/SnZrMoP that the material is made up of aggregates and agglomerates of spherical nano-particles. Presence of some rod shape particles in the nano-composite and their presence and their formation by the nucleation of nano-particle is very clear in HR-TEM images of the nano-composite material.
HR-TEM images of the nano-composite shows a network of spherical particles and on the other hand, Shows the fusion of some nanoparticles to generate some rod shapes particles of size range ≈ 40â??90 nm., these rod shape transformations is more prominent. The selected area diffraction (SAED) pattern of nano-composite PTD/ SnZrMoP diffraction rings which are in line with the peaks obtained in its XRD spectra and is pointing towards its semi crystalline profile.
The XRD-spectra of nano-composite PTD/SnZrMoP show some broad, low intensity peaks at lower 2θ values, whereas some prominent, sharp peaks can also be seen at higher 2θ values, which might be due to the incorporation of the organic moiety into the inorganic counterpart. All these observations are pointing towards semi-crystalline nature of nano-composite ion exchanger.
To find out the selectivity of the exchanger for a particular ionic moiety. The distribution studies were performed in the solutions of 14 different metal ions in four distinct solvent mediums [19]. The order of selectivity coefficients in aqueous media is: Hg2+ (1000) > Co2+ (362.50) > Cr2+ (264.70) > Pb2+ (137.80) > Ca2+ (133.00) >
Zn2+ (60.82) > Cd2+ (60.00) > Fe3+ (52.78) > Cu2+ (42.61) > Mg2+
(28.80) > Ba2+ (27.74) > Sr2+ (13.88) > Al3+ (6.84) > Ni2+ (4.59).
From the value of distribution coefficient the exchanger was observed to be selective for Hg (II) ions, whereas the rest metal ions were poorly absorbed. Thus, from the above observations, it is clear that the nano-composite ion exchanger PTD/SnZrMoP can be utilized for the separation of Hg (II) ions from the waste water sample collected from different industrial units and as an analytical application, it can also be used as a promising agent for the fabrication of Hg (II) ion selective electrode.
Physio-chemical properties of the membrane based on nano- composite PTD/SnZrMoP
On the basis of ion exchange capacity sample K-5 was considered best for the synthesis of ion exchange membrane. For the optimization of the membrane material, the epoxy resin and nano-composite PTD/ SnZrMoP were mixed in ratios, 1:9, 2:8, 3:7 and 4:6 (w/w). All these
fabricated membranes were tested for the properties like: thickness, water content, porosity, IEC, swelling, etc., and the best membrane is considered one which should have high value of IEC and higher strength. That the membrane M-3 has been considered fit for further studies due to its better IEC, moderate thickness and moderate porosity. The higher ion exchange capacity of membrane M-3 may be due to the proper distribution of resin particles in the binder phase.
To study the distribution of nano-composite material in binder phase field emission scanning electron microscopy of the membrane based on NEAT-EP (EP-stands for epoxy resin) and membrane based on nano-composite (M-3) was performed. Represents the surface view of the membrane based on NEAT-EP, which is clearly demonstrating the presence air pockets and pores on the membrane surface. In the cross-section view of membrane based on NEAT-EP, the number of air pockets and pores are almost negligible, which supports its impermeable nature. The texture of the inner core of the membrane based on EP is smooth and layered. The surface view of the membrane based on PTD/SnZrMoP nano-composite shows the even distribution of nano-composite material in the binder phase. The cross section view of the composite membrane based on PTD/SnZrMoP shows the distribution of pores and air pockets in its interior structure of membrane to facilitate the exchange process. The cross section view also depicts the rough texture composite membrane
TG/DTA curves of SnZrMoP (inorganic counterpart) and PTD/ SnZrMoP (nano-composite) respectively, shows the comparison between their thermal studies. It has been concluded from the above investigations that the total weight loss between the temperature range of 50 °C to 150 °C is only due to the loss of external water molecules and water of crystallization from the inorganic and nano-composite ion exchanger both, the weight loss between the temperature range 150 to 350 °C, in both the cases is due to the conversion of inorganic phosphate into pyrophosphate [31]. While in case of nano-composite the weight loss between 350 to 750 °C temperature range might be due to the decomposition of organic polymer [19]. Clearly demonstrated the high thermal stability of nano-composite PTD/SnZrMoP ion exchanger than its inorganic counterpart SnZrMoP up to the temperature range of 350-550 °C, the weight loss is only due to the partial decomposition of Poly-o-toluidine.
TG/DTA curves for the membrane based on NEAT-EP. The figure demonstrated that the % age weight loss below 150 °C is 1.46
%, which might be due to the loss of interstitial water molecules, the weight loss is very low in this temperature zone which predicts the hydrophobic nature of membrane based on epoxy resin. The other important prediction from the thermogram of membrane based on NEAT-EP is that, the degradation of epoxy resin starts at 350 °C and almost completed at ≈500 °C. The % age weight loss between this temperature range is 83.90 %, which might be due to the cabonization of hydrocarbonated compounds. In contrast to the EP-NEAT membrane the membrane based on PTD/SnZrMoP nano-composite ion exchanger the weight lost below 150 °C is 4.09 % that may be due to the loss of interstitial water present in binder phase and resin phase, while the weight loss between 150 to 350 °C is 19.044 %, which might be due to the decomposition of epoxy resin and conversion of inorganic phosphate into pyrophosphate [31], this change was confirmed from the endothermic hump in the same region of DTA curve. The weight loss between a temperature range of 350 to 500
°C is 43.455% that may be due to the decomposition of epoxy resin and organic counterpart of nano-composite (PTD). Represents the comparison between the thermal studies of the membrane based on
NEAT-EP and membrane based on PTD/SnZrMoP nano-composite ion exchanger. The thermogram of membrane based on nano- composite materials shows three plateau areas which confirm the three phase transition in membrane material. Three endothermic humps in same region temperature zone support this transformation.
Electrode Characteristics
The fabricated electrode was tested for various parameters like response time, the slope of the calibration curve, lower detection limit, linear response concentration range, effective pH range etc. The following observation were obtained from the investigation of these studies: the response time of ion selective electrode was evaluated as a function of time. The response time of Hg (II) ISE based on nano- composite was observed to be 16 seconds.
Potential measurements were made for different concentrations of the Hg (II) ions ranging from 1×10â??10 M to 1×10â??1 M, i.e. almost tenfold dilution of stock solution for test purpose and 5×10â??2 M solution was poured inside the electrode tube. The observed values of electrode potential were plotted against the log of the activities of Hg (II) ions. The reproducibility of electrode system was checked by repeating the same experiment three times. The limit of detection of calibration curve was calculated according to IUPAC recommendation
[32] from the point of intersection of two extrapolated lines of curves as reported in literature [33-35]. The lower detection limit of proposed electrode was observed to be 3.55×10â??8 M and the slop of the curve was observed to be ‘Sub-Nernstion’, being 24.82 mV/decade for Hg
(II) ions. The Non-Nernstian behavior of the electrode might be due to the different activities of ions in solution and the phase boundary of electrode. Similar behavior was observed by Jain [36], Harsanyi et al. [37,38], Kaushal et al. [14]. The proposed electrode exhibits the comparable linear range over the concentration 1×10â??7 M to 1×10â??1 M.
To see the effect of change in concentration of the an internal Hg (II) ion solutions on the slope of the calibration curve of ISE. The value of electrode potential obtained is plotted against the log of activity of Hg (II) ion solution by using 1×10â??2 and 1×10â??3 M internal solutions one by one. The observed results show that the variation in the concentration of internal solution has no significant effect on the response of the proposed electrode.
The the slope of the calibration curve was also investigated in partially non-aqueous media. The solutions were prepared by adding acetone and ethanol mixed water to the Hg (II) ion solutions. The proposed sensor was investigated by using 1×101 to 1×10-10 M analyte solution concentrations. The electrode potential obtained is plotted against the log of activity of Hg (II) ion solution. It was observed that the slope of the calibration curve remains unchanged even in the presence of non-aqueous solvents. It has been observed that this ISE can be used successfully even non aqueous solvents.
The work ability of electrode was also tested by changing the pH value of analyte solution, the pH dependence was tested by using 1×10â??3 M Hg (II) ion solution in the pH range of 1.0 to 13.0. The observations., can be simplified as, the value of membrane potential remains almost constant in the pH range of 2.5 to 8.0. The sharp rise in electrode potential value at pH below 2 may be due to the response of the electrode towards both mercuric (Hg2+) and hydronium ions (H3O+) present in the medium, whereas the sharp decline in electrode potential value above 8 pH is due to the precipitation of Hg
•ions as Hg (OH)2 [14].
The analytical importance of proposed membrane electrode has been established by making its use as an indicator electrode for potentiometric titration of Hg(NO3)2 (1.0×10â??2 M) solution against EDTA (1.0×10â??2M) as titrant. The observed electrode potential values were plotted against the volume of EDTA solution used. From the resultant plot it has been concluded that the value of electrode potential keeps on decreasing on addition of standard EDTA solution due to the non-availability of free Hg (II) ions.
This property, which is designated as the single, most important characteristic of any ISE electrode. This property is used to check the selectivity of any proposed electrode for particular ions even in the presence of a large number of interfering ions. The selectivity coefficient K_(A,B)^POT was calculated by using the fixed interference method FIM [23] at 1×10-4 M concentration of the interfering ions. It has been observed from the results that the value of K_(A,B)^POT < 1 for all the interfering ions. So, from the above observations, it can be concluded that the proposed electrode is highly selective for Hg (II) ions than other interfering ions present in aqueous medium.
When not in use for more than one day, the electrode was kept stored in distill water. The lifetime of the proposed electrode was tested to be 4 months. Within that limit of time there was no significant change in response time and slop of the proposed electrode. The leaching of the membrane material with test solutions might be responsible for the possible change in response time and slop of the electrode. Potential responses were recorded for different concentrations of Hg (II) ion solutions after the interval of 15, 30, 60, 90 and 120 days. No significant variation was observed in response time and slope of calibration curves. It was also observed that calibration curves retain the near Nernation value even after 20, 60 and 120 days.
The response properties of the proposed Hg (II) ion selective electrode was compared with the other reported Hg (II) ion selective electrodes. The linear response of proposed electrode is in the concentration range of 1.0×10â??7â??1.0×10â??1 M and shows the sub Nernation slop of 24.82 mV/decade, which is better than other reported electrodes. The lower detection limit for the proposed electrode is 3.55×10-8 M, it also shows better working pH range, the response time of the electrode is 16 second, which is less than the response time of most of the reported electrodes. The other positivity of the proposed electrode is that it can also be used effectively with non-aqueous solvents, whereas none of other reported electrode was used in non-aqueous solvents.
The practical utilization of the ISE was also investigated through its use in the potentiometric estimation of Hg (II) ions in the waste water samples collected from different industrial units. First the Hg2+ ions were estimated in the water samples through simple complexometric titrations by making the use of PAN-(1-(2-Pyridylazo)-2-naphthol) indicator and a buffer solution of pH ≈ 3. The % age error was observed to be 6%, In the second step the same titrations were performed by the use of fabricated Hg (II) ion selective electrode based on nano- composite PTD/SnZrMoP as an indicator electrode, the % age error in this case has been reduced to 4% only. The experiment was repeated thrice to check the validity of proposed electrode.
The nano-composite PTD/SnZrMoP material was also used for the separation of Hg2+ ions from the water samples collected from different industrial units. The effluent samples were collected
from: CFL unit, thermometer unit and thermal power plant. After purification and neutralization the samples were passed through the column of PTD/SnZrMoP and were eluted with 1.0 M HNO3.
It is evident from observed results that the separation of Hg (II) ions from the industrial effluents was quite sharp and the metal ions recovery was above than 90%. Thus, the prepared nano-composite and fabricated electrode has a promising future for the estimation and separation of traces of Hg II) ions in various industrial effluents.
Conclusion
The present study is based on the fabrication, characterization and applications of Hg (II) ion selective electrode based on nano-composite PTD/SnZrMoP. The response time of the proposed electrode has been observed to be 16 second, which is less than the response time of most of the reported electrodes. The other positivity of the proposed electrode is that it can be used most effectively even in non-aqueous solvents. Whereas none of other reported electrode was used in non-aqueous solvents. The slope of the electrode is Non- Nernation and its lower detection limit is 3.55×10-8 M. The electrode follows the Nernation response over a wide concentration range (1.0×10- 7â??1.0×10-1 M). As an analytical application the electrode was used for the potentiometric estimate the Hg (II) ions in the industrial effluents. Thus the nano-composite cation exchanger PTD/SnZrMoP can find a place in various industries dealing with separation processes.
Acknowledgement
The authors gratefully acknowledge Sri Guru Granth Sahib World University, Fatehgarh Sahib, Punjab (India) and Guru Nanak Dev Polytechnic College, Ludhiana (India) for providing the necessary facilities.
References
- Loomis D, Grosse Y, Lauby-Secretan B, Ghissassi FE, Bouvard V (2013). The carcinogenicity of outdoor air pollution. Lancet Oncol, 14(13), 1262–1263.
[Crossref] [Google Scholar] [Indexed]
- Hunter D, Bomford RR, Russel DS (1940). Poisoning by methylmercury compounds. Quart. J. Med, 9(3), 193-226.
[Crossref] [Google Scholar] [Indexed]
- Khan MDA, Akhtar A, Nabi SA, Khan MA (2014). Synthesis, characterization, and photocatalytic activity of polyaniline-Sn (IV) iodophosphate nanocomposite: its application in wastewater detoxification. Ind Eng Chem Res, 53(39) (2014)15253â??15260.
[Crossref] [Google Scholar] [Indexed]
- Heitland P, Koster HD (2006). Biomonitoring of 37 Trace Elements in Blood samples From Inhabitants of Northen Germany by ICP-MS. J. Trace Elem. Med. Biol., 20 (2006) 253â??262.
[Crossref] [Google Scholar] [Indexed]
- Wen X, Wu P, Xu K, Wang J, Hou X (2009). On-Line Precipitation–Dissolution in Knotted Reactor for Thermospray Flame Furnace AAS for Determination of Ultratrace. Microchem J, 91 (2), 193-196.
[Crossref] [Google Scholar] [Indexed]
- Ullah MR, Haque ME (2009). Spectrophotometric Determination of Toxic Elements (Cadmium) in Aqueous Media. J Chem Eng, 25(2009), 1â??12.
- Bonfil Y, Brand M, Kirowa-Eisner E (2000). Trace Determination of Mercury by Anodic Stripping Voltammetry at the Rotating Gold Electrode. Anal. Chem. Acta, 424 (2000) 65â??76.
- Kamel NHM, Sayyah EM, Abdel-al AA (2011). Removal of lead, cobalt and manganese from aqueous solutions using a new modified synthetic ion-exchanger. Arch. Appl. Sci. Res., 3 (2) (2011) 448â??464.
- Han WS, Wia KC, Parkb WS, Honga TK (2012). Mercury ion selective poly (aniline) solid contact electrode based on 2-mercaptobenzimidazol ionophore. Russ. J. Electrochem., 48 (5) (2012) 525â??231.
- Mahajan RK, Kaur I, Lobana TS (2003). A Mercury (II) Ion-Selective Electrode Based On Neutral Salicylaldehyde Thiosemicarbazone. Talanta, 59(1), 101-105.
- Chai G, Yuan YR, Dai J (2006). A Mercury (II) Ion-selective Electrode Based on N, N-Dimethyl formamide-salicylacyl hydrazone as a Neutral Carrier. Anal Sci, 22(4), 579-582. [Crossref]
- Kaushal S, Badru R, Kumar B, Mittal SK, Singh P (2016). Fabrication of a mercury (II) ion selective electrode based on poly-o-toluidine–zirconium phosphoborate. RSC Adv, 6 (4), 3150-3158.
[Crossref] [Google Scholar] [Indexed]
- Khan AA, Paquiza L (2011). Characterization and ion-exchange behavior of thermally stable nano-composite polyaniline zirconium titanium phosphate: Its analytical application in separation of toxic metals. Desalination, 265(1-3), 242–254.
- Khan AA, Inamuddin (2006). Applications of Hg (II) sensitive polyaniline Sn (IV) phosphate composite cation-exchange material in determination of Hg2+ from aqueous solutions and in making ion-selective membrane electrode. Sens Actuators B Chem, 120(1), 10-18.
[Crossref] [Google Scholar] [Indexed]
- Khan AA, Inamuddin, Akhtar T (2008). Organic-inorganic Composite Cation-exchanger: Poly-o-toluidine Zr (IV) Phosphate-based Ion-selective Membrane Electrode for the Potentiometric Determination of Mercury. Anal Sci, 24(7), 881-887.
[Crossref] [Google Scholar] [Indexed]
- Valsaraj PV, Janardanan C (2013). Separation of Toxic Metal Ions Such as Pb (II), Cu (II) and Hg (II) from Industrial Waste Water Using Newly Synthesized tin-zirconium molybdophosphate Cation Exchanger. J Environ Nanotechnol, 2(2013), 6-14.
- Kaur R, Kaushal S, Singh PP (2019). Efficient removal of Hg (II) ions from waste water by a new nano-composite poly-o-toluidine tin-zirconium (IV) molybdophosphate. Int J Environ Anal Chem, 1-16.
[Crossref] [Google Scholar] [Indexed]
- Kaur R, Kaushal S, Singh P (2019). Selective removal of lead (II) ions and estimation of Ca (II) ions using poly-o-toluidine-zirconium (IV) molybdophosphate. Chem Papers, 73(8), 2027-2039.
[Crossref] [Google Scholar] [Indexed]
- Pungor F (1997). How to understand the response mechanism of ion selective electrodes. Talanta, 44(9), 1505−1508.
[Crossref] [Google Scholar] [Indexed]
- Nabi SA, Naushad M, Bushra R (2009). A new hybrid EDTA-zirconium phosphate cation- exchanger: synthesis, characterization and adsorption behavior for environmental monitoring. Ads Sci Tech, 27(4), 423â??434.
- Sadjadi MS, Khalilzadegan A (2015). The effect of capping agents, EDTA and EG on the structure and morphology of CdS nanoparticles. J Nano-Oxide Glasses, 7(4), 55-63.
- Valsaraj PV, Janardanan C (2015). Polyaniline embedded tin zirconium tungstate composite ion exchange material for the removal of heavy metal ion and organic pollutant from aqueous solution. Mater Sci Ind J, 13(9), 277-288.
- Janardanan C, Valsaraj PV (2014). Investigation on polyaniline enriched tin cerium phosphate thermally stable fibrous composite ion exchanger for environmental remediation. Int J Adv Chem, 2(1), 6-11.
[Crossref] [Google Scholar] [Indexed]
- Nabi SA, Bushra R, Khan AM (2010). Synthesis, characterization and analytical applications of a new composite cation exchange material poly-o-toluidine stannic molybdate for the separation of toxic metal ions. Chem Eng J, 165(2), 529-536.
[Crossref] [Google Scholar] [Indexed]
- Khan AA, Sheen S (2014). Chronopotentiometric and electroanalytical studies of Ni (II) selective polyaniline Zr (IV) molybdophosphate ion exchange membrane electrode. J. Electroanal. Chem., 714-715 (2014) 38-44.
[Crossref] [Google Scholar] [Indexed]
- Sharma G, Pathania D, Naushad M, Kothiyal NC (2014). Fabrication, characterization and antimicrobial activity of polyaniline Th (IV) tungstomolybdophosphate nanocomposite material: Efficient removal of toxic metal ions from water. Chem Eng J, 251(1), 413–421.
[Crossref] [Google Scholar] [Indexed]
- Nabi SA, Naushad M (2008). Synthesis, characterization and analytical applications of a new composite cation exchanger cellulose acetate-Zr (IV) molybdophosphate. Colloids Surf A Physicochem Eng Asp, 316(1-3), 217-225.
- Bakker E (1997). Selectivity of Liquid Membrane Ion-Selective Electrodes. Electro analysis, 9(1), 7-12.
[Crossref] [Google Scholar] [Indexed]
- Mittal SK, Sharma HK, Kumar SKA (2006). Synthesis Characterization of Zirconium (IV) Antimonoarsenate as Potentiometeric Sensor. React Funct Polym, 66(10), 1174â??1181.
- Ganjali MR, Nozouri P, Alizhadeh TA, Tajrodi Y (2007). Fabrication of Highly Selective and Sensitive Gd (III)-PVC Ion Membrane Sensor. Sens Actuators B Chem, 120(2), 487-493.
[Crossref] [Google Scholar] [Indexed]
- Mittal SK, Sharma HK (2004). Samarium (III) Selective Membrane Sensor Based on Tin (IV) Boratophosphate. Sensors, 4(8) 125-135.
- Jain AK, Singh RP, Bala C (1982). Solid Membranes of Copper hriacyanoferrates (III) as Thallium (I) Sensitive Electrode. Anal Lett, 15(1982) 1557â??1563.
- Harsanyi EG, Toth K, Polos L, Pungor E (1982). Adsorption Phenomenon of Silver Iodide Based Ion Selective Electrodes. Anal Chem, 54(7), 1094-1097.
- Harsanyi EG, Toth K, Pungor E (1984). The Behaviour of Silver Sulphide Precipitate Based Ion Selective Electrode in Low Concentration Range. Anal Chem Acta, 161(1984), 333â??341.
[Crossref] [Google Scholar] [Indexed]
- Abu-Shawish HM (2009). A mercury (II) selective sensor based on N, N′-bis (salicylaldehyde)-phenylenediamine as neutral carrier for potentiometric analysis in water samples. J Hazard Mater, 167(1-3), 602-608.
[Crossref] [Google Scholar] [Indexed]
- Perez-Marin L, Otazo-Sanchez E, Macedo-Miranda G, Avila-Perez P, Chamaro JA, Lopez-Valdivia H (2000). Mercury (II) ion-selective electrode. Study of 1, 3-diphenylthiourea as ionophore, Analyst, 125(10) (2000)1787â??1790.
- Mashhadizadeh MH, Sheikhshoaie I (2003). Mercury (II) ion-selective polymeric membrane sensor based on a recently synthesized Schiff base. Talanta, 60(1), 73-80.
[Crossref] [Google Scholar] [Indexed]
- Abbas II (2012). Mercury (II) selective membrane electrode based on calix [2] thieno [2] pyrrole. Int J Chem, 4(1), 23â??29.
[Crossref] [Google Scholar] [Indexed]