Research Article, J Pharm Drug Deliv Res Vol: 11 Issue: 1
Implications of Circadian Dosing- Time in the Chrono tolerance and the Rhythmic Delivery of the 5- Fluorouracil (5-Fu) in Female Rats and its Relevance to Cancer Therapeutics
Ines Bouali1, Ichrak Dridi2,3*, Wafa Gadacha1, Sonia Zaeid4, Naceur Boughattas2, Mossadok Ben Attia1, Karim Aouam3, Abdelaziz Souli1
1Department of Sciences, Environmental Biomonitoring Laboratory, University of Carthage, Zarzouna, Tunisia
2Department of Medicine, Laboratory of Pharmacology, University of Monastir, Monastir, Tunisia
3Department of Clinical Pharmacology, CHU Fattouma Bourguiba de Monastir, University of Monastir, Tunisia
4Department of Medical Oncology, CHU Fattouma Bourguiba in Monastir, Monastir, Tunisia
*Corresponding Author:Ichrak Dridi
Laboratory of Pharmacology, Faculty of Medicine, University of Monastir, 5019 Monastir, Tunisia,
Tel: +216 97 66 37 54;
E-mail: dridi.ichrak@yahoo.fr
Received date: 06 December, 2021, Manuscript No. JPDDR-22-51081;
Editor assigned date: 08 December, 2021, Pre QC No. JPDDR-22-51081 (PQ);
Reviewed date: 20 December, 2021, QC No. JPDDR-22-51081;
Revised date: 30 December, 2021, Manuscript No. JPDDR-22-51081 (R);
Published date: 06 January, 2022, DOI:10.4172/2325-9604.1000202
Citation: Dridi I, et al. (2021) Implications of Circadian Dosing-Time in the Chrono tolerance and the Rhythmic Delivery of the 5-Fluorouracil (5-Fu) inFemale Rats and its Relevance to Cancer Therapeutics. J Pharm Drug Deliv Res 11:1.
Abstract
The present work aims to study whether the tolerance to 5-fluorouracil (5-FU) varies according to the circadian dosing timein female rats.The influence of dosing-time on tolerance to 5-FU was investigated in 120 adult female Wistar rats. All animals weresynchronized under a light-dark cycle (12:12). A potentially
lethal dose of 5-FU was first determined to be 350 ± 0.92mg/kg, it was administrated by intraperotonial route (i.p.). 5-FUwas injected to a total of 90 female rats divided in six circadianstages [1, 5, 9, 13, 17 and 21 Hours After Light Onset (HALO)]. Three toxicity end-points were analyzed such us survival rate,body weight and rectal temperature. Drug dosing at 21HALO resulted in 100% survival rate as compared to drug dosing at 5HALO (0%). The Cosinor analysis revealed a significant circadian rhythm in survival with an acrophase at 21.81HALO ±0.68 hours (p<0.0002). The most rectal temperature changeand body weight occurred when 5-FU was injected at 5 HALO resulted in -61.89% weight loss whereas drug dosing at 21HALO was a weight gain 11.39% (Ø=2 HALO ± 1.39 hours, p<0.0033).
Keywords: 5-fluorouracil; Circadian rhythm; Tolerance; Survival; Rectal temperature
Introduction
At the beginning of the seventies, chronopharmacology became a scientific discipline thanks to A. Reinberg and F. Halberg. It is defined as the study of variations in the effects of drugs according to the time of administration. This approach allows therapeutic optimization of anticancer agents. Indeed, it is a science that constitutes an indispensable experimental step to guide the realization of chronotherapy protocols as it is the case for anticancer drugs [1,2].
Life is organized not only in space but also in time [3]. Biological rhythms have been demonstrated in all the processes associated with malignant transformation of cells as well as in the cellular proliferation of healthy and tumorous tissues [4]. In the 1950s, researchers discovered the existence of spontaneous and rhythmic variations in numerous physiological functions [5]. These circadian rhythms play a role whose respective importance is for each drug. It is therefore advisable to adjust our habits such as taking medication in accordance with our internal clock in order to improve our vitality and health [6]. The administration of a drug at the right biological time in relation to circadian rhythms can lead to a modulation of the toxicity of this drug [7,8]. These data could be indispensable in the clinic as they allow for treatment modification to increase the efficacy of a drug or decrease its side effects through the regulation of homeostatic systems such as sleep and activity, hormone levels, appetite, and other bodily functions with 24 hour cycles [9]. In addition, this hierarchical organization of the circadian system affects the temporal control of human physiology at the organ and whole body level [10,11]. A hypothalamic central clock called the Suprachiasmatic Nuclei (SCN) with significant external influence from the light-dark cycle, feeding behavior, and social patterns are responsible for organizing this system and as a result the functioning of the organs optimally. The circadian clock gene Bmal1 results in activation of p53, a pro-apoptotic molecule known to play an important role in cell apoptosis, a process that involves several rhythmic components. In addition, p53 expression and apoptosis were decreased by circadian rhythm disruption due to Per2 mutation, Per1 knockout or chronic time-lag. There is also a circadian effect on BCL-2 and BAX, signaling molecules implicated in apoptosis [12-18].
In the treatment of cancer, the development of new therapeutic and clinical methods to mask adverse effects and deliver them as well as the development of new methods of delivery to tissues is essential. However, several fascinating modern treatment strategies and drugs have been explored and evaluated, 5-Fluoracil (5-FU), a pyrimidine anti metabolite, an anti-cancer drug has been widely studied since its discovery in 1957 [19,20] as a rationally designed anticancer agent [21,22]. 5-FU is a prodrug who's a drug that undergoes biotransformation before manifesting its therapeutic effect [23,24], used in the treatment of cancer which has been found particularly in the treatment of colorectal, gastric, breast and head and kidney cancer [25-29]. However, 5-FU shows a risk for patients who do not tolerate this drug by the appearance of more or less serious effects [30]. A 1987 follow-up study with an additional 30 patients receiving postoperative chemo radiotherapy shows that adjuvant chemo radiotherapy with 5-FU improved the mean 2-year overall survival rate (25 vs. 10.9 months) and (46% vs. 18%)[31]. 5-FU has limitations such as a short organic half-life due to rapid metabolism of 10-20 minutes in plasma [32], incomplete oral absorption due to metabolism by dehydrogenated dihydropyrimidines in the bone marrow and gastrointestinal tract, and non-selective action on healthy cells [33,34] and other health problems such as alopecia, vomiting and diarrhea [20,35,36]. However, most chemotherapy drugs have negative impacts and studies are underway to minimize their side effects [37]. In theory, the timing of drug administration could increase the effectiveness of pharmacotherapeutic outcomes and as a goal to provide guidance for potential chronotherapy. Dispite its introduction more than 30 years ago, 5-FU has not yet been studied from a chronopharmacological point of view.
In the current study, animal training was confirmed using rectal temperature as a marker rhythm. Circadian rhythm temperature as well as body weight measurement are one of the most important toxicity criteria in animal model research due to its reliability [38,39].
To demonstrate that the iterative 24-hour rhythm may influence the biological tolerance to drugs used in chemotherapy such as 5-Fluoracil in breast cancer, we studied the variation of the tolerance of this anticancer agent at different circadian stages (1, 5, 9, 13, 17 and 21 Hours After the Onset of Light -HALO). This study will allow to eventually determine the optimal time(s) of drug administration.
Materials and Methods
Animals and synchronization
The study involved 120 female Wistar rats (Pasteur Institute of Tunis, 1002 Belvédère, Tunis, Tunisia), aged ten to twelve weeks and having a similar body weights. The animals were housed by 4 to 5 per cage with free access to a standard diet and water ad libitum in a chronobiological animal facility for at least 3 weeks before the start of the experiments [40]. This self-contained chronobiological facility is equipped with a temperature control that is maintained at 22 ± 2°C and relative humidity 50%-60% as well as light intensity at the cage level ranging from 223 lux to 315 lux (white light, neon tube type, Phillips). Monitoring of the reversed light regime using a Gossen-Panlux electronic luxmeter held at the level of the cage lid and perpendicular to the incident beam of the light source, and the distribution of the animals in the two separate rooms within the animal house, allows the exploration of 6 circadian stages (1, 5, 9, 13, 19, 21 HALO) during the usual daytime work. The synchronization consists in alternating 12 hours of light (L) and 12 hours of darkness (D) (Light: 7:00 hours-19:00 hours (L/D: 12/12) and darkness: 19:00 hours-07:00 hours). All manipulations during the dark phase were performed under a dim red light (7 lux). All the experiments were carried out in accordance with the directives on the care and use of the laboratory animals in accordance with the standards carried out in accordance with the ethical recommendations of the Tunisian Association of the Science of the Laboratory Animals (ATSAL), which adheres to the International Committee on Laboratory Animal Science (ICLAS). The control of the rhythmicity of the rectal temperature (using a thermometer) is a simple and non-traumatic method to check the synchronization of the rats more precisely the acrophase (peak hour) being used as index of marker rhythm (Boughattas et al.).
Drug
Fluorouracil (5-FU) is a soluble pyrimidine analogue with anticancer action having the molecular formula C4H3FN2O; its chemical name is 2,4(1H,3H)â??pyrimidinedione, 5â??fluorouracil its molecular weight is 130.08 g/mol. It is an injectable solution. The product is supplied in a clear glass vial with a clear plastic (polyethylene terephthalate) ONCO-TAIN® sheath to protect the vial from breakage. It was provides from a pharmaceutical company Cytopharma S.A. (Zaghouan, Tunisia). The dose used for toxicity study was 350 mg/kg. Each dose was administered intraperitonally (i.p.) to animals.
Study Design
A preliminary study was conducted to determine the lethal dose inducing 50% (LD50) of lethality in female rat.
Different doses 5-FU were prepared following a geometric progression with a ratio of 1.2 between each two successive doses. These doses of drug were administrated by i.p. route at 11:00h local time, i.e., 4 HALO (rest span) to minimize the influence of circadian changes.
The LD50 was estimated from curves expressed as percentage of mortality, who is converted to a probit scale, against the log-dosage of 5-FU according to the application of the Miller and Tainter method.
A total of 120 female rats were treated by i.p. route at six different circadian stages (1, 5, 9, 13, 17, and 21 HALO). The 30 control rats (five rats/time point) received a saline injection (NaCl 0.9%). The 90 rats in the MMF-treated group (15 rats/time point) were injected a potentially lethal dose 350 mg/kg of body weight, which corresponds to the LD50. Mortality was recorded there after daily throughout a subsequent 30-day span. Survival rate was determined for each group at the end of the study. Rectal temperature and body weight were measured every two days for the two-week span following treatment to explore the three end-points of toxicity. Body weight was measured with a high precision balance (Radwag, WPS 360/C/2). Body weight loss is expressed in percentage relative to the initial body weight prior to treatment. Rectal temperature was measured with a digital thermometer (OMRON Ecosmart, Holt 55005).
Data Analysis
In the acute lethal toxicity tests, four different doses were used as recommended by Miller and Tainter. The LD50 was estimated from the graphs of percent (%) mortality converted to probit as a function of logarithmic dose of 5-FU. The probit 5 being equal to 50%. LD50 was expressed as Mean ± SEM values (mg/kg). Means (m) and Standard Error of the Mean (SEM) were calculated for each study variable. Significant differences in quantitative data between experimental groups were performed by Analysis of Variance (ANOVA) which allowed to confirm the rhythmicity detected by the chronogram.
In order to check the synchronization of the animals and analyze the time series, the Cosinor least squares based method was used for a 24 hour rhythm with a test period t ≈ 24 hours. For a given period, a rhythm is characterized by 3 parameters:
- The mesor (M) which is the adjusted mean of the 24 hour rhythm.
- The amplitude (A) which is half the difference between the maximum and minimum values of the derived cosine curve.
- The acrophase (Ø) which is the peak time (with the onset of the light). Knowing that the onset of the light 0 HALO being used as a phase reference [41].
When the confidence limits of these three parameters result in a percentage of 95%, the rhythm is considered statistically significant. Indeed, the confidence limit was calculated by computer log-probit analysis. In principle, a rhythm is detected when A is non-zero (non-zero magnitude verified by the F-test of variance represented by a straight line fit of the time series data at p<0.05) [42]. The significance of the statistics conventional and chronobiological statistics was necessary to qualify the temporal changes as rhythms.
Results
Animals and synchronization
The synchronization of the animals was validated by a stable photoperiodic regime that is verified at the end of the second week by a 24-hour circadian rhythm following the Cosinor method (with p <0.0001). Indeed, the Cosinor test shows that the acrophase of this 24 hour rhythm occurred in the mid-half of the dark-activity span (Ø =18.48 HALO ± 0.33 h). Knowing that, the acrophase allows the estimation of the best chronotolerance to 5-FU. The characteristics of the 24-hour pattern in rectal temperature confirmed the physiological entrainment of female rat to the environmental LD schedule. The circadian acrophase of rectal temperature is used as a marker for the dosing time dependent differences in VPA associated toxicity (Figure 1).
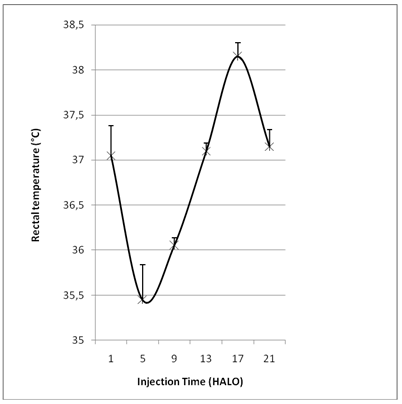
Figure 1: Variation of temperature (°C) according to the circadian time before treatment.
Rectal temperature
The circadian rhythm in rectal temperature was maintained stable in control rats during the two weeks of the study 5-FU induced a significant hyperthermia on days 3, 5, 7 and 9 of +0.67, +0.94, +1.39 and +1.48°C respectively.
The temperature increase varied as a function of both drug dosing time (+4°C at 5 HALO and +0.45°C at 13 HALO) and sampling day (one-way ANOVA with p<0.004) (Figure 2). During the third week, the rectal temperature values were similar to those of the controls on day 1. The circadian rhythm of temperature was altered he became an ultradian rhythm with 8 hours.
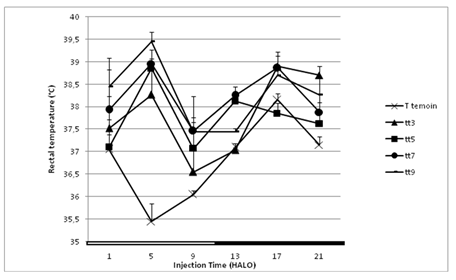
Figure 2: Rectal temperature (°C) variation according to the circadian time (1, 5, 9, 13, 17 and 21 HALO) of 5-FU administration (350 mg/kg, i.p.) in a total of 90 female rats on day 1 before treatment, and on days 3, 5, 7 and 9 following treatment in surviving animals. Differences related to both dosing-time and sampling-day were statistically validated by one-way ANOVA (p ≤ 0.01).
Body weight changes
A significant mean body weight loss (denoted by a negative sign) in 5-FU-treated rats was observed (ANOVA; p<0.01) as compared to the controls (%).
The mean of body weight losses were -5.53 on day 3, -18.29 on day 5, -13.04 on day 9, -13.72 on day 11 and -9.73 on day 13. Body weight decreased significantly until day 5 which corresponded the maximum loss (-18.29%), then all live female rats began to regain body weight from the second week after treatment (-9.71% on day 13). Body weight loss varied according to dosing- time of 5-FU treatment (Figure 3).
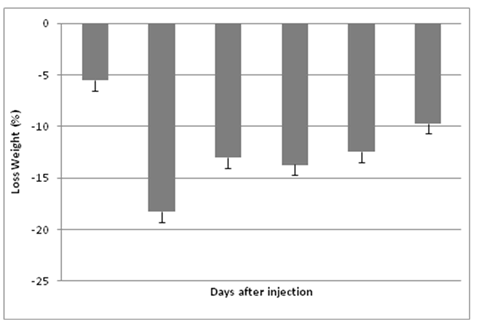
Figure 3: Body weight loss variation (%) according to the days after VPA-administration in a total of 90 mice. Results are expressed as mean percent change ± SEM. and were statistically validated by one-way ANOVA (p ≤ 0.01).
It was maximal when the drug was injected at 5 HALO (-61.89%) whereas we have observed a weight gain of 11.39% in the group treated at 21 HALO, i.e. during activity-dark span. The difference between peak-trough was statiscally significant. A circadian body weight loss rhythm (p<0.0033) was detected by Cosinor with 53.30% of percent rhythm. At the end of the experiment, all surviving animals recovered their body weight (Figure 4).
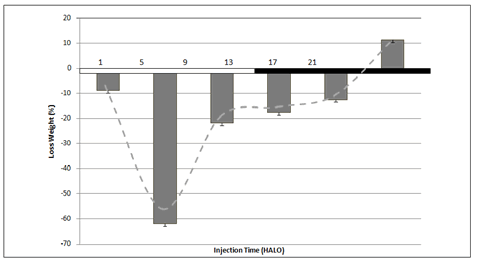
Figure 4: Body weight loss variation (%) according to the circadian dosing time of 5-FU in a total of 90 rats. Di�erences between mean values of the highest and lowest body weight loss were statistically validated by ANOVA (p<0.001).
Survival rate
Mortality was observed between the third and the ninth day following 5-FU treatment. All the animals, which died, suffered from bloody diarrhea, vomiting, eye disorders (excessive tearing, conjunctivitis) and hair loss. Subsequently, no death occurred until day 30 when the study was completed.
Over all dosing times, the mean survival rate was 43%. The maximum (100%) and minimum (0%) survival rates occurred when 5-FU was administered at 21 HALO (end of the dark-activity span of female rats) and 5 HALO (Figure 5). The difference being validated by the Cosinor analysis. A circadian rhythm in survival was detected by cosinor (p<0.0002) with an acrophase was localized at 21.08 HALO ± 0.68 hours.
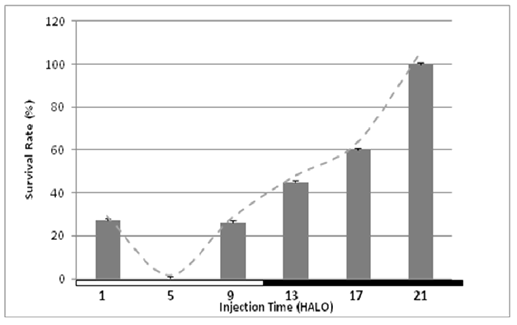
Figure 5: Circadian variation in tolerance to 5-FU (350 mg/kg) as assessed by survival rate (%) at each of six circadian dosing times (1, 5, 9, 13, 17, and 21 HALO) in 90 rats. The optimal drug tolerance occurred when the drug was injected in the end of dark phase (during the animals’ activity phase).
Discussion
The chronopahramcological approach is based on the determination of the most appropriate time(s) of the day for the administration of a potentially toxic drug in order to achieve a rational or even optimal chronotherapy and thus improve the tolerance of the treatments [10]. This is the main objective of this article.
The circadian rhythm of rectal temperature is a marker of synchronization in rodents [38,40,43,44] because of its cyclic periodicity which is quite stable regardless of the time of the year. Indeed, the phase and period of this rhythm seem to faithfully reflect the variations of the light-dark alternation. It is an endogenous rhythm of sinusoidal shape and high amplitude located in the middle of the nocturnal activity phase where it presents the highest activity of the animal [8]. The animal models used are often rodents (mouse, rat) where the consumption of water and food during the dark phase is one of the main factors of this peak in circadian temperature [45].
Numerous animal experiments as well as clinical studies have provided strong evidence that drug side effects can be modified by circadian time and/or the timing of drug administration within the 24 hours of a day [46]. To this end, we first investigated the appropriate time(s) of best tolerance to 5-FU in rodents by monitoring rectal temperature, body weight loss, and survival after treatment with the anticancer agent at all six circadian stages (1, 5, 9, 13, 17, 21 HALO).
Determination of the LD50 at a fixed cycle time is a relevant method to measure the short-term toxic potential (acute toxicity) of this drug whose mean value was estimated to be 349.86 ± 0.92 mg/kg. The administration of the LD50 of 5-FU reveals several signs of toxicity such as: rapid breathing, eye irritation in some, weight loss and diarrhea probably associated with gastrointestinal toxicity. Mortality occurred after 14 days. Experiments that were done on a dog show that 5-FU has side effects on bone marrow stem cells and epithelial cells of the intestinal crypts and a disruption of the intestinal mucosa are the main sites of toxicity of this anticancer agent [47].
Treatment with a toxic dose (equivalent to LD30) of 5-FU at different circadian stages did not cause a disruption of the circadian rhythmicity of rectal temperature. Acrophases were always located in the middle of the active phase (18 HALO) and troughs were located around the middle of the resting phase. During all days, there is no significant difference in Mesor and amplitude. On the other hand, a statistically significant hyperthermia was observed in animals treated at the 5 HALO stage with +4°C and at the 13 HALO stage with +0.45°C. This shows that the administration of a single dose of 350 mg/kg b.w. causes a dephasing of the circadian rhythm.
On the other hand, the loss of body weight showed a disruption of circadian rhythmicity with a shift of acrophase towards the beginning of the dark phase. Indeed, the results of 5-FU administration showed a loss of body weight in all circadian stages, this loss being statistically significant on the 13th day with (-9.71%) following the injection. It is maximal at the time of 5 HALO with (-61.89%) and minimal at the 21 HALO with 11.39%. Similarly, the loss of body weight shows a disruption of circadian rhythmicity with a shift of acrophase towards the middle of the resting phase. Regarding the other two survival variables (survival rate and survival time), the results showed a maximum at the 21 HALO stage with a percentage of 100% and a minimum of 0% from 5 HALO.
A study by Cuba et al. carried out on male mice of the strain CF-1, in which the batch of positive controls received a dose of 5-FU (60 mg/kg/D) showed a loss of weight at both times of the experiment or that was significant compared to the other groups. antineoplastic treatments are responsible for the loss in body weight [47,48]. Another study that was done on hamsters shows that 5-FU can cause oral mucositis.
However, an experimental study in dogs revealed the toxicity induced by 5-FU. The experiment was based on the oral consumption of 5-FU cream at doses ranging from 6 to 271 mg/kg (but no animal could resist a dose higher than 43 mg/kg). Generally, clinical signs appeared after 45 to 60 minutes and death occurred after 6 hours to 16 hours of ingestion. The main clinical signs are grand mal seizures, tremors, dyspnea, cyanosis, vomiting, diarrhea, hypersalivation. The survival rate is estimated at 39%. These variations in the documented results in dogs from our studies are based on differences in several factors such as the anatomy of the animals, the sex and the route of administration of the drug and the concentration.
From the circadian variation of the studied variables: Temperature, body weight and survival rate, it can be said that the optimal tolerance to 5-FU occurs when this drug is injected in the second half of the activity phase of rats (21 HALO). In contrast, minimal tolerance is observed when 5-FU is administered at 5 HALO.
The serious consequences of 5-FU call for new research of new therapeutic targets. The understanding of its biopathology is of growing interest for emerging studies.
In conclusion, in order to better carry out chronotherapy protocols with the anticancer agent 5-FU in human subjects, it is necessary to highlight the rhythms of tolerance and efficacy on a biological scale.
References
- Boughattas NA, Li XM, Filipski J, Lemaigre G, Filipski E, et al. (1996) Modulation of cisplatin chronotoxicity related to reduced glutathione in mice. Hum Exp Toxicol 15: 563â?? [Crossref], [Google Scholar], [Indexed]
- Lévi F (2006) Chronotherapeutics: The relevance of timing in cancer therapy. Cancer Causes Control 17: 611â?? [Crossref], [Google Scholar], [Indexed]
- Dijk DJ, Lockley SW (2002) Integration of human sleep-wake regulation and circadian rhythmicity. J Appl Physiol 92: 852â?? [Crossref], [Google Scholar], [Indexed]
- Focan C (2002) Chronobiological concepts underlying the chronotherapy of human lung cancer. Chronobiol Int 19: 253â?? [Crossref], [Google Scholar], [Indexed]
- Kotz SA, Ravignani A, Fitch WT (2018) The Evolution of rhythm processing. Trends Cogn Sci 22: 896â?? [Crossref], [Google Scholar], [Indexed]
- Nahmias Y, Androulakis IP (2021) Circadian effects of drug responses. Annu Rev Biomed Eng 23: 203â?? [Crossref], [Google Scholar], [Indexed]
- Ben-Cherif W, Gharbi R, Sebai H, Dridi D, Boughattas NA, et al. (2010) Neuropharmacological screening of two 1,5-benzodiazepine compounds in mice. C R Biol 333: 214â?? [Crossref], [Google Scholar], [Indexed]
- Khedhaier A, Attia MB, Gadacha W, Sani M, Bouzouita, et al. (2003) Circadian rhythms in toxic effects of the serotonin antagonist ondansetron in mice. Chronobiol Int 20: 1103â?? [Crossref], [Google Scholar], [Indexed]
- Ohdo S (2010) Chronotherapeutic strategy: Rhythm monitoring, manipulation and disruption. Adv Drug Deliv Rev 62: 859â?? [Crossref], [Google Scholar], [Indexed]
- Levi F, Schibler U (2007) Circadian rhythms: Mechanisms and therapeutic implications. Annu Rev Pharmacol Toxicol 47: 593â?? [Crossref], [Google Scholar], [Indexed]
- Lévi F, Okyar A, Dulong S, Innominato PF, Clairambault J (2010) Circadian timing in cancer treatments. Annu Rev Pharmacol Toxicol 50: 377â?? [Crossref], [Google Scholar], [Indexed]
- Jiang W, Zhao S, Jiang X, Zhang E, Hu G, et al. (2016) The circadian clock gene Bmal1 acts as a potential anti-oncogene in pancreatic cancer by activating the p53 tumor suppressor pathway. Cancer Lett 371: 314â?? [Crossref], [Google Scholar], [Indexed]
- Gery S, Komatsu N, Baldjyan N, Yu A, Koo D, et al. (2006) The circadian gene per1 plays an important role in cell growth and DNA damage control in human cancer cells. Mol Cell 22: 375â?? [Crossref], [Google Scholar], [Indexed]
- Granda TG, Liu XH, Smaaland R, Cermakian N, Filipski E, et al. (2005) Circadian regulation of cell cycle and apoptosis proteins in mouse bone marrow and tumor. FASEB J Off Publ Fed Am Soc Exp Biol 19: 304â?? [Crossref], [Google Scholar], [Indexed]
- Filipski E, Innominato PF, Wu MW, Li XM, Lacobelli S, et al. (2005) Effects of light and food schedules on liver and tumor molecular clocks in mice. J Natl Cancer Inst 97: 507â?? [Crossref], [Google Scholar], [Indexed]
- Fu L, Pelicano H, Liu J, Huang P, Lee C, et al.(2002) The circadian gene Period2 plays an important role in tumor suppression and DNA damage response in vivo. Cell 111: 41â?? [Crossref], [Google Scholar], [Indexed]
- Carrillo E, Navarro SA, Ramirez A, Garcia MA, Grinan-Lison C, et al. (2015) 5-Fluorouracil derivatives: A patent review (2012-2014). Expert Opin Ther Pat 25: 1131â?? [Crossref], [Google Scholar], [Indexed]
- Jubeen F, Liaqat A, Sultan M, Iqbal SZ, Sajid I, et al. (2019) Green synthesis and biological evaluation of novel 5-fluorouracil derivatives as potent anticancer agents. Saudi Pharm J 27: 1164â?? [Crossref], [Google Scholar], [Indexed]
- Álvarez P, Marchal JA, Boulaiz H, Carrillo E, Velez C, et al. (2012) 5-Fluorouracil derivatives: A patent review. Expert Opin Ther Pat 22: 107â?? [Crossref], [Google Scholar], [Indexed]
- Heidelberger C, Chaudhari NK, Mooren D, Griesbach L, Duschinsky R, et al. (1957) Fluorinated pyrimidines, a new class of tumour-inhibitory compounds. Nature 179: 663â?? [Crossref], [Google Scholar], [Indexed]
- Goodman DW (2007) Lisdexamfetamine dimesylate: The first prodrug stimulant. Psychiatry (Edgmont) 4: 39â??
- Hashimoto Y, Yoshida H, Yamada T, Aisu N, Yoshimatsu G, et al. (2020) Current status of therapeutic drug monitoring of 5-fluorouracil prodrugs. Anticancer Res 40: 4655â?? [Crossref], [Google Scholar], [Indexed]
- Longley DB, Harkin DP, Johnston PG (2003) 5-Fluorouracil: mechanisms of action and clinical strategies. Nat Rev Cancer 3: 330â?? [Crossref], [Google Scholar], [Indexed]
- Meyerhardt JA, Mayer RJ (2005) Systemic therapy for colorectal cancer. N Engl J Med 352: 476â??[Crossref], [Google Scholar], [Indexed]
- Rich TA, Shepard RC, Mosley ST (2004) Four decades of continuing innovation with fluorouracil: Current and future approaches to fluorouracil chemoradiation therapy. J Clin Oncol 22: 2214â?? [Crossref], [Google Scholar], [Indexed]
- Šuleková M, Váhovská L, Hudák A, Žid L, ZeleÅ?ák V (2019) A study of 5-fluorouracil desorption from mesoporous silica by RP-UHPLC. Molecules 24: 1317. [Crossref], [Google Scholar], [Indexed]
- Wang X, Lin J, Zhang X, Liu Q, Xu Q, et al. (2003) 5-Fluorouracil-cisplatin adducts with potential antitumor activity. J Inorg Biochem 94: 186â?? [Crossref], [Google Scholar], [Indexed]
- Goirand F, Lemaitre F, Launay M, Tron C, Chatelut E, et al. (2018) How can we best monitor 5-FU administration to maximize benefit to risk ratio?. Expert Opin Drug Metab Toxicol 14: 1303â?? [Crossref], [Google Scholar], [Indexed]
- Keene KS, Rich TA, Penberthy DR, Shepard RC, Adams R, et al. (2005) Clinical experience with chronomodulated infusional 5-fluorouracil chemoradiotherapy for pancreatic adenocarcinoma. Int J Radiat Oncol Biol Phys 62: 97â?? [Crossref], [Google Scholar], [Indexed]
- Altinok A, Lévi F, Goldbeter A (2009) Identifying mechanisms of chronotolerance and chronoefficacy for the anticancer drugs 5-fluorouracil and oxaliplatin by computational modeling. Eur J Pharm Sci 36: 20â?? [Crossref], [Google Scholar], [Indexed]
- Arias JL, López-Viota M, Delgado AV, Ruiz MA (2010) Iron/ethylcellulose (core/shell) nanoplatform loaded with 5-fluorouracil for cancer targeting. Colloids Surf B Biointerfaces 77: 111â?? [Crossref], [Google Scholar]
- Kevadiya BD, Patel TA, Jhala DD, Thumbar RP, Brahmbatt H, et al. (2012) Layered inorganic nanocomposites: A promising carrier for 5-fluorouracil (5-FU). Eur J Pharm Biopharm 81: 91â?? [Crossref], [Google Scholar], [Indexed]
- Miura K, Kinouchi M, Ishida K, Fujibuchi W, Naitoh T, et al. (2010) 5-fu metabolism in cancer and orally-administrable 5-fu drugs. Cancers 2: 1717â?? [Crossref], [Google Scholar], [Indexed]
- Radwan AA, Alanazi FK (2014) Design and synthesis of new cholesterol-conjugated 5-Fluorouracil: A novel potential delivery system for cancer treatment. Molecules 19: 13177â?? [Crossref], [Google Scholar], [Indexed]
- Zhang Q, Xu Y, Lv J, Cheng M, Wu Y, et al. (2018) New utilization of polygonum multiflorum polysaccharide as macromolecular carrier of 5-fluorouracil for controlled release and immunoprotection. Int J Biol Macromol 116: 1310â?? [Crossref], [Google Scholar], [Indexed]
- Ben-Cherif W, Dridi I, Aouam K, Ben-Attia M, Reinberg AA, et al. (2012) Chronotolerance study of the antiepileptic drug valproic acid in mice. J Circadian Rhythms 10:3. [Crossref], [Google Scholar], [Indexed]
- Nelson W, Scheving L, Halberg F (1975) Circadian rhythms in mice fed a single daily meal at different stages of lighting regimen. J Nutr vol 105: 171â?? [Crossref], [Google Scholar], [Indexed]
- Reinberg A, Smolensky M (1983) Chronobiology and thermoregulation. Pharmacol Ther 22: 425â?? [Crossref], [Google Scholar], [Indexed]
- Edwards MC (1970) Foot-and-mouth disease in the brown rat. J Comp Pathol 80: 543-548. [Crossref], [Google Scholar], [Indexed]
- Sani M, Sebai H, Boughattas NA, Ben-Attia M (2011) Time-of-day dependence of neurological deficits induced by sodium nitroprusside in young mice. J Circadian Rhythms 9: 5. [Crossref], [Google Scholar], [Indexed]
- Boughattas NA, Levi F, Hecquet B, Lemaigre G, Roulon A, et al. (1998) Circadian time dependence of murine tolerance for carboplatin. Toxicol Appl Pharmacol 96: 233â?? [Crossref], [Google Scholar], [Indexed]
- Ohdo S, Ogawa N, Song JG (1995) Chronopharmacological study of acetylsalicylic acid in mice. Eur J Pharmacol 293: 151â?? [Crossref], [Google Scholar], [Indexed]
- Wanner PS, Primola Gomes TN, Pires W, Guimaraes JB, Hudson ASR (2015) Thermoregulatory responses in exercising rats: methodological aspects and relevance to human physiology. 2: 457-75. [Crossref], [Google Scholar], [Indexed]
- Ohdo S, Watanabe H, Ogawa N, Yoshiyama Y, Sugiyama T (1996) Chronotoxicity of sodium valproate in pregnant mouse and embryo. Jpn J Pharmacol 70: 253â?? [Crossref], [Google Scholar], [Indexed]
- Sayre RS, Barr JW, Bailey EM (2012) Accidental and experimentally induced 5-fluorouracil toxicity in dogs. J Vet Emerg Crit Care 22: 545â?? [Crossref], [Google Scholar], [Indexed]
- Arribas L, Hurtos L, Taberna M, Peiro I, Vilajosana E, et al. (2017) Nutritional changes in patients with locally advanced head and neck cancer during treatment. Oral Oncol 71: 67â?? [Crossref], [Google Scholar], [Indexed]
- Lin MC, Shueng P, Chang WK, Chang PMH, Feng HC, et al. (2018) Consensus and clinical recommendations for nutritional intervention for head and neck cancer patients undergoing chemoradiotherapy in Taiwan. Oral Oncol 81: 16â?? [Crossref], [Google Scholar], [Indexed]
- Skeff MA, Brito GAC, de Oliveira MG, Braga CM, Cavalcante MM, et al. (2014) S-nitrosoglutathione accelerates recovery from 5-Fluorouracil-induced oral mucositis. PLoS ONE 9: e113378. [Crossref], [Google Scholar], [Indexed]