Research Article, J Nucl Ene Sci Power Generat Technol Vol: 11 Issue: 9
Increasing the Energy in the Building Envelope Using Phase Change Materials
Nouhaila Benachir*, Taoufiq Mouhib and Farida Bendriaa
1Department of Physic engineering, Hassan first University of Settat ENSA Berrechid LISA Laboratory Morocco
*Corresponding Author: Nouhaila Benachir, Oncology Clinic Department of Physic engineering, Hassan first University of Settat ENSA Berrechid LISA Laboratory Morocco E-mail: n.benbachir@uhp.ac.ma
Received date: 29 August, 2022, Manuscript No. JNPGT-22-73579; Editor assigned date: 31 August, 2022, Pre QC No. JNPGT-22-73579 (PQ); Reviewed date: 14 September, 2022, QC No. JNPGT-22-73579; Revised date: 21 September 2022, Manuscript No. JNPGT-22-73579 (R); Published date: 28 September, 2022, DOI:10.4172/2325-9809.1000292
Citation: Benachir N, Mouhib T, Bendriaa F (2022) Increasing the Energy in the Building Envelope Using Phase Change Materials. J Nucl Ene Sci Power Generat Technol 11:9.
Abstract
The overall objective of the project is to improve the living conditions of the local population. The specific objective is to set up a pilot unit using local building materials, with low environmental impact, provided with organic phase change materials. The research work will lead to innovation, through the combination of local materials and biomaterials based PCM, and will allow building materials companies to develop by improving their products a complete numerical model of the zone will be developed using the TRNSYS 204 simulation tool. Our research strategy aims to examine the PCM use as thermal energy storage materials for the building sector .and also verify the performance of PCM as a new way to stabilize the air temperature and control the comfort inside the buildings envelope. The study includes a modelling and numerical simulation to verify with experimental components. The simulation was carried out for the climate zone of Morocco. The results of the simulation showed that the use of PCM in building envelope, decreasing the relative humidity and ambient temperature , saving total energy demand and consumption for heating and cooling than building envelope without PCM all months of the year. The article can be helpful for the building energy efficiency, thermal performance and the goal of (building plus +) zero and net zero energy.
Keywords: Comfort; TRNSYS; Phase change material;
Thermal energy storage; Building envelope
Introduction
These phase change materials are often used to store energy to overcome times of mismatch between thermal supply and demand in a building, such as storing solar thermal energy for space heating in the evening. The lifting of all energy subsidies by 2025 in Morocco will exacerbate the energy disparity. Reducing energy costs is a major challenge in Morocco. This mobilization is justified primarily by the costs associated with energy consumption. Indeed, 10% of Moroccan gross domestic expenditure in 2014 was dedicated to energy expenditure. In addition, such consumption has disastrous environmental consequences in the long term: greenhouse gas emissions, depletion of natural resources, etc. Among the sources of energy consumption, heating and air conditioning systems represent about 50% of the total expenditure in buildings. This considerable proportion can be explained in part by the great temperature variations recorded in Morocco, which make the contribution of air-conditioning or heating indispensable almost at all times. The development of the green buildings passes thus inevitably by an optimization of the use of the systems Heating and air conditioning. In this perspective, a clear difference between the use of systems during the day and during the night has been observed. In fact, during the day, the solar irradiation incident on the facade of a building coupled with the various internal gains (occupancy density, lighting, etc.) density, lighting, etc.), cause a high demand for air conditioning. Conversely, during the night, the internal and solar gains are solar gains are non-existent and the outside temperature is lower: heating is often required. From this point of view, storing the excess loads emitted during the day and re-emitting them during the night when heating is required is an ideal solution for increasing energy efficiency. Indeed, less heat Most materials used in buildings have either a relatively low thermal mass or a high structural mass, which means that they can be used in a variety of ways. or a high structural mass, such as concrete. The provision of thermal inertia is therefore necessary, but is accompanied by disadvantages and restrictions in terms of structural design, aesthetics and ecology. However, there is a type of high thermal inertia and excellent specific properties, which is becoming more and more important in the design of new Phase Change Materials (PCMs). The high thermal inertia of these materials stems from their ability to change phase at a useradjustable temperature .Depending on the properties of the thermal zone where we are, it is therefore possible to integrate PCMs and to optimize their parameters in order to favorably dephase the energy consumption peaks and energy consumption and, by the same token, significantly reduce the use of the heating and air conditioning system. Consequently, the integration of PCM in the envelopes of new buildings or in renovation would contribute to reduce the energy bill in the building sector in building sector in Morocco. The envelope of traditional buildings has remained for a long time a passive means to limit heat loss or heat input and to ensure a certain air conditioning by its thermal inertia. Indeed, the thermal energy stored in the walls during the hot periods is restored during the cold periods. One of the key objectives of the research on low energy buildings is to find a way to manage the time differences between energy sources and energy consumption. The main objective here is to model, quantify and optimize the impact of the presence of PCMs in a thermal zone conditions of Morocco with the ultimate goal of developing low energy consumption buildings. Energy consumption. First, a complete numerical model of the thermal behavior of a zone will be developed using the simulation tool Trnsys, with the possibility of including phase change materials. The achievement of this objective will allow to have at disposal a customized code, fully scalable, able to calculate all the thermal variables of interest in a building in interaction of interest in a building in interaction with the environment. Such a complex wall technology integrating PCM must be properly taken into account in order to simulate the energy behavior of buildings and to evaluate their impact in several domains (environmental, thermal behavior of buildings, etc.) Once the thermal characterization of the building has been completed, the second specific objective will be to process all of the data obtained in order to measure the impact of the presence of CAMs from several perspectives. On the one hand, the average temperature will be examined after integration of the PCMs in a zone.
The most effective method is using PCMs in both cooling and passive and active of the building. The ways by which the PCM are incorporated, namely the direct incorporation, immersion and encapsulation have been analyzed by Hawes and Feldman [1]. Soaresa and al [2]. Provided a comprehensive review of the previous research addressing the passive latent heat thermal energy storage systems with PCM in buildings and their related performance.
The simulation of a PCM is supported by type 204. The simulations are performed for different indoor convective heat transfer coefficients (h-value), where the h-value increases from 0.5 to 10 W/m2.K. The simulation, results show that the heating energy demand increases when the h-value increases, but the cooling load decreases slightly. Bontemps [3] showed the new PCM module was validated with experimental. The results showed that, during the summer, there is a reduction of 2°C in indoor temperature for the room with PCM walls compared to the room without PCM walls. It was also shown that, in winter, the thermal discharge for the wall with PCM in the interior temperature drops to -9°C Xu, Zhang [4] further investigated the effect of various parameters such as, melting temperature, the heat of fusion and thermal conductivity of PCM on the thermal performance of the building. They found that the heat of fusion and thermal conductivity of PCM should be greater than 120 kJ/kg and 0.5 W/(mK) a large number of numerical studies, which have been recently performed in different countries, helped in better understanding of the physics behind the PCM-enhanced building products and their potential energy performance. For decades, different types of PCM-enhanced building boards and plasters have been the most popular objects of computer simulations. Earliest numerical studies started during the late 1970and had been continued till the1990s. They were mainly focused on gypsum wallboards impregnated with paraffin (Solomon [5]; Tomlinson and Heberle [6]; Kedl [7]; Stovall and Tomlinson [8]; Kissock [9]). A combined experimental–numerical work was performed by Athienitis [10], who conducted extensive field testing followed by one-dimensional numerical analysis of a full-scale outdoor test hut with PCM-enhanced gypsum board installed as an inside wall sheathing. In more recent projects, PCM wallboards and plasters containing microencapsulated PCMs (Hawlander [11]; Darkwa and Callaghan [12], Schossig [13]; Kendrick and Walliman [14] have been studied. In addition, the thermal performance of shapestabilized PCM board products has been analyzed using numerical methods (Kuznik [15]; Virgonet [16]; Constantinescu [17]. Due to flammability concerns about paraffinic PCMs, a number of numerical models have been utilized recently to analyze the thermal performance of boards and insulation products thermally enhanced with bio-based alternatives kinds to paraffin(Rozanna [18]; Riza [19]; Kośny [20] Dhanusiya and Rajakumar [21].This work consists of the thermal simulation in dynamic mode, using TRNSYS [22] software to compare the thermal behavior of a building with walls incorporating bricks and air-core with walls incorporating Phase Change Materials (PCM). The simulation was carried out for the climatic zone of (Casablanca Nouasseur) (Figures 1-3).
Methodology
Mathematical description
If the controller the PCM wall model on if
γi=1 and ΔtL ≤ (th - tl), γo=1 If γι=1 and ΔtL > (th-tl), γo=0
if the controller the PCM wall model of γi=0 and Δth ≤ (th - tl), γo=1
The heating and air conditioning (on/off) Temperature th'=th1+( γ1· ΔTdb)–( γset· ΔTset).
2 heat source thl2'=th2 + γ2·( ΔTdb – (γset· ΔTset)).
Conditioning source: tc'=tc–(γ3· ΔTdb). And ySet (any) Set point for the controlled variable
When the 1 stage source temperature t h is greater than or equal to t min, the first stage enable function γe is set to 1. Otherwise, γe=0. The controller the PCM wall then functions as shown in the diagram.
The Energy rate mhCp and temperature ti qL=(ua (tb- ta)- qgain) are applicator in the controller PCM wall if > 0 0 otherwise (Tables 1 and 2).
Materials | Tickness (cm) | Density (kg/m3) | Resistance (mk/w) | Heat capacity (J/(kg·K)) | Heat conductivity coefficient (W/(m·K)) |
---|---|---|---|---|---|
Brick layer | 25 | 1800 | 0.337 | 1000 | 0.88 |
Air gap | 4 | 1000 | 0.278 | 1200 | 0.09 |
Brick layer | 7 | 1800 | 0.079 | 1000 | 0.88 |
PCM layer | 5 | 850 | 0.106 | 2200 | 0.47 |
Table 1: Thermo physical properties of materials.
NTYPE# | Label | Description | Unit |
---|---|---|---|
NTYPE 62 | PMV | Predicated Mean vote (PMV) value of zone | |
NTYPE 63 | PPD | Predicated Percentage of Dissatisfied persons (PPD) Value of Zone | (%) |
Table 2: Zone outputs.
qt=ql if qL≤ εcmin(ti-tb) Equal 0
If qL ≤ ε cmin(ti - tb) 0 otherwise q aux=0 if ql ≤ ε cmin (ti - tb) ql or to=ti-qt/mhCp if qt > 0 t i otherwise.
lhr=latent load ACHRAES regalement (latent )/(sensible) ratios of about
The general total and latent air conditioning loads are calculated as qcool=qsens /(1lhr)
qlat=qcool-qsens floating building construction temperature, No Auxiliary the qsolar=qsolar total+qsolar adjacent-qblk_ref-qblk_absorbqblk_ esh-qsolar_los qsolarabci- qblk_risw-qish_cciqsolarwgain( kJ/hr)
• qsolar total: Total external solar on all windows of the building structure
• qsolar adjacent : The extern solar energy due to exchange with adjacent wall. ( Losses ,mold - gains)
• qblk: Solar blocked
• qblk_esh : The extern solar blocked due to external shading devices of all windows of a zone
• qblk absorb: Solar blocked due to absorption on building structure differential equation for changing internal energy is determinant dt=γ ε Cmin ti - tb + qgain - ua (tb - ta + qaux - qsens ).
where, γ=1 if mi > 0 0 otherwise qsens=(1- lhr) . qcool this differential equation is solved for the final building temperature for each time step, trf and tr using qt=γ . ε *cmin (ti - tR).
ql=ua (tr - ta)-qgain, qlat=qcondito–qsens
• cmin: Minimum capacitance rate of the building construction heat exchanger
• cp: The capitance heat of the flow
• lhr: Ratio of latent heating
• qaux :The auxiliary energy
• qconditio: air conditioning rate of cooling
• qgain: Heat gains
• ql: The rate heat from the internal building structure
• qlat rate at which air conditioning input is used to minimize the building structure relative humidity
• qsens rate at which air conditioning input is used to minimize the building structure internal temperature
• Ta: The ambient temperature
• Ti: The internal temperature
• Tb: The building temperature
• ε: Effectiveness of the building heat exchanger
• Δt: Simulation time
• qblk_risw: Solar blocked due to reflection on internal shading devices of all windows
Energy race for internal surface: Energy_Surface=-(dq wall)dt +qcomo-qcomi+ qt_rgain_i +qt_rgain_w - qt_al.
• ( dqwall) dt: Change of surface and internal energy
• qcomi : Exchange heat flux to inside zone.( Equipment , people)
• qcomo : Exchange heat flux to outside zone (going to outside-; going into wall +)
• qtrgain_i: The total radiative gains for internal surface
Input of walls: The parameters and proprieties about the wall. the input of zone represent the information about the wall , the user can be allow the box in the upper provides an overview of all parameters walls and add, delete or edit the walls of a zone, of clicking on a wall within this overview box (Figures 4 and 5).
Cooling air conditioning: The air conditioning requirement (Figure 6) of any wall of the zone subject to idealized to controlling the air conditioning requirement and also can be representing by specifying a cooling type. So when the air conditioning equipment is modeled in (Multi Building) the external TYPE 56 component; the air conditioning requirement type should not be used [23-26]. Like that the ventilation, relative humidity and temperature should be defined as inputs, fed by outputs from the conditioning equipment component(s) or negative transfers change radiative, convective and conductive gains should be defined. The specification of a air conditioning control is optional and the default setting of the a air conditioning control is off.
Gains internal: Total gains including by the people the internal wall (Table 3), internal working electrical equipment, computer, etc. The information about the specification gains is not obligator (Figures 7-12).
Material | Wall+PCM204 | Wall+PCM | Wall-PCM |
---|---|---|---|
Tout,casa min(°C) | 18.79 | 18.79 | 18.79 |
Tin,min(°C) | 22.96 | 25.4 | 26.55 |
ΔT(°C) | 4.17 | 6.606 | 7.71 |
Tout,casa Max(°C) | 30 | 30 | 30 |
Tin,Max(°C) | 23.92 | 25.69 | 27.08 |
ΔT(°C) ΔT (wall+PCM)and (wall-PCM) :3.17°C | 6.08 3.17 | 4.31 1.4 | 2.92 |
Table 3: Minimum and Maximum amplitudes of the external and internal ambient temperature.
Figures 13-15 and Table 4 show the minimum and maximum magnitude of the internal ambient temperature without phase change materials and with phase change materials, 204 and external temperature. The building envelope with phase change materials debilitate the temperature of 3.17°C in comparison to the building without PCM. The PCM phase change materials can attenuate the internal temperature to 11.4% of thermal debilitate reduction. The employment of phase change material increases the internal temperature of the building envelope .So the internal temperature in the building envelope has inferior to than the ambient temperature extern , which is close by the restrict of thermal comfort space [27-29].
Material | Wall+PCM204 | Wall-PCM |
---|---|---|
Tout,casa min(°C) | 15 | 15 |
Tin, min(°C) | 18.2 | 18.4 |
ΔT(°C) | 3.2 | 3.7 |
Tout, casa Max(°C) | 29 | 29 |
Tin, Max(°C) | 24 | 27.4 |
ΔT(°C) (wall+PCM)and (wall-PCM) : 3.4°C | 5 | 1.6 |
Table 4: Minimum and maximum debilitate of the extern and inner surface temperature.
The growth rate of the outside and inside air temperature in the building envelope in CASALANCA MOROCCO, with PCM and that without PCM. The simulation convey that the extern air temperature varies between 16°C and 28°C. The inside air temperature of the building envelope without phase change materials varies in the middle 21°C and 27°C, which is under than that of the outside air. The air temperature in the building envelope with phase change materials varies in the middle 20°C and 24°C. So the phase change materials play the role as an organic insulating material that bring down the heat transfer and the air temperature by 3°C, 55% of the relative ratio humidity. The deference temperatures are higher and therefore closer to the thermal comfort.
Relative humidity (RH) ( FIG 17.22)
The results conveyed that the building with phase air gap and phase change materials not only had the reaction on the heat flow transfer and temperature and exchange transfer convection ,conduction and radiative transfer in the wall, but also had reaction on the ratio relative humidity and moisture transfer in the wall. The apex relative ratio humidity and the moisture flux of the wall with phase change materials were both small. Compared with external relative ratio humidity and the relative ratio humidity of the wall without phase change materials. The reduction of the relative humidity of the PCM wall is 28.6 % with the external relative ratio humidity and the relative humidity percentage range of the wall without PCM was 13%. Although the apex diminution of the relative humidity was small, it is concluded that the risk of crystallization, condensation and infiltration of the wall could be diminish Figure 16.
Thermal comfort:
The "PMV" index (Figure 17) gives the average vote of the individuals surveyed which indicates opinions on their average thermal sensations according to the ASHRAE scale1 which varies from (-3) to (+3) where each number expresses a thermal sensation.
In our case the value of PMV with PCM varies between 1 and -1 in the summer period. The negative values express very low temperatures and the positive values indicate very high temperatures. The thermal comfort zone is between (-1) and (+1). An index of the PMV equal to (0) expresses an optimal feeling of thermal comfort (Figures 18-20).
Energy requirements for heating and cooling
Figures 21-23 shows the annual evolution of the sensible energy at the inner surfaces of the building envelope in CASABLANCA .The wall with phase change materials minimize the energy by more 16.8%. In this case, the phase change (Table 5) material function as an insulating material that dominate the heat flow about sensible energy transfer, and diminish the heat exchange between the indoor and the outdoor spaces.
Consumption of air conditioning KWH | Heating+PCM KWH | Colling+PCM KWH | Heating-PCM KWH | Colling-PCM KWH | SOMME (H+C+PCM) KWH | SOMME (H+C-PCM) KWH | Gains KWH | Reduction% |
---|---|---|---|---|---|---|---|---|
consumption (KWH) | 870 | 60 | 1200 | 132 | 930 | 1332 | 402 | 30.18% |
Consumption(KWH/m2) | 12.44 | 17.36 | 13.31 | 5.85 | 28%heating 52%colling |
Table 5: Energy consumption of the building envelope.
n=(1-Q+pcm/Q-pcm)*100 Q+pcm=930kwh Q-pcm=1332kwh n=30.18 %
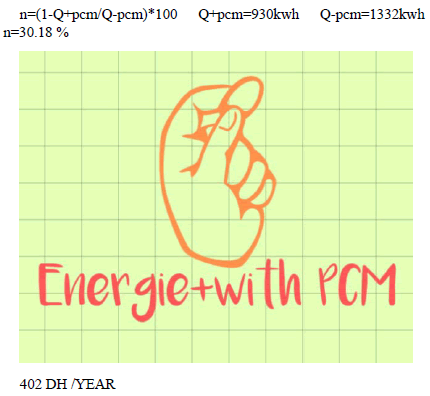
Summarizes the annual heating and air conditioning loads. The total thermal and electrical energy consumption for heating and air conditioning equipment’s shows that the building with PCM minimize the energy for the tow loads compared to a building without PCM , the energy need for the PCM wall is 1125 KJ and without PCM 1348 KJ difference of 226 KJ . SO the building envelope with phase change materials maintain the thermal and electrical energy consumption17%. The energy consumption with PCM in August was 33 Kwh and without pcm51 Kwh. The some results convey that the presence of the phase change material in the building envelope maintain the annual consumption and demand for heating and cooling and achieved energy savings by diminishing the cold period retaining the heat solar charging during the phase change can storage the solar energy (Figure 24) and heat transfer and minimize the period of air conditioning maximally avoiding overheating due to solar gains and internal gains and control the heat flux and thermal comfort in the interior wall for the building envelope . The annual needs for consumption air conditioning and heating are according to the NOUSSEUR climate zone 1 (AGADIR), these annual needs are 25 kWh/m2/year for residential buildings (RTCM, 2014). The results clearly convey that the charge needs are about 13.31 kWh/m²/year.These percentage needs represent 48.76% of the needs set by the MOROCCAN regulation.
The comparison of the convective solar heat flow asserts that the phase change materials has a effect influence on the reduction of energy solar heat gains transmitted in the interior of the building envelope, which directly affects the heat transfer convection conduction and radiative transfers, the air temperature variations and the thermal comfort and the relative humidity of the building as well as its improving the energy performance.
The improvement of the wall through the reduction of the thermal transmission also influences in a positive way the internal ambient temperature, the creation of thermal comfort inside the building and present the best inertia for the all wall the building envelope.
Figure 25 shows the results of the growth of the internal surface temperature without and with PCM phase change materials in summer and the outside surface temperature without and with phase change materials. The internal surface temperature of phase change materials is 23°C and without PCM 25.3°C, within a fluctuation range of 2.06°C. The outside surface temperature of phase change materials is 29°C and without PCM 34.42°C within a fluctuation range of 5.36°C .so the phase change materials acts the control for the external and intern surface temperature and thermal and electrical energy and thermal and fresh comfort zone.
Conclusion
The application the composite Phase Change Materials (PCM) into the building envelope results in an increase in the electrical and thermal energy storage capacity, providing an effective and reliable means of improving the energy efficiency of buildings. It is concluded that composites in corporating phase change materials are capable of diminish the thermal and electrical energy costs, about air conditioning and heating demands of the building. Also we can contribute to diminish the CO2 emissions associated with air conditioning and heating equipment. The thermal and electrical energy performance of a building located in (CASABLANCA NOUASSEUR) were addressed. First, a dynamic thermal simulation of the building using TRNSYS TYPE 204 software was according and its results were successfully validated against the experimental results obtained from the monitoring. In this study, the effects of the location of the incorporating theses phase change materials on the thermal and electrical performance of the multi-layer wall3D is studied numerically under the climatic conditions of (CASABLANCA NOUASSEUR). The simulation also convey that the use of phase change materials in brick walls diminish overheating in the summer period, losing the ambient indoor air temperature by 3.4°C in summer, require the annual percentage about the electrical and internal energy for heating and air conditioning by 31%. These percentage needs represent 48.76% of the needs set by the MOROCCAN regulation (RTCM.2014). So the total results convey that the presence of the phase change material in the building envelope maintain the annual consumption and demand for heating and cooling and achieved energy savings by diminishing the cold period retaining the heat solar charging during the phase change can storage the solar energy and heat transfer and minimize the period of air conditioning maximally avoiding overheating due to solar gains and internal gains and control the heat flux and thermal comfort in the interior wall .In summer, a high thermal capacity prevents the indoor air temperature from rising, minimizes the cooling load or eliminates it altogether and minimizes the investment costs of the necessary cooling equipment. It is concluded that the efficiency of the PCM can be improved if the building can be properly ventilated so for that in the next article we will deal with the integration of mechanic solar ventilation with the phase change material to increase the thermal and electrical energy and created the comfort and fresh zone and attenuate the performance energy to achieve a positive building+
Acknowledgement
First, I sincerely thank the technical inputs of Mr Mouhib Taoufiq and Bendriaa Farida of Physical Engineering Department are acknowledged and HassanI First University of Settat, (ENSA) Ecole Nationale des Sciences Appliquées, LISA Laboratory and CNRST organism.
References
- Hawes DW, Feldman DD, Banu D (1993) Latent heat storage in building materials. Energy and Buildings 20: 77-86 77.
- Soares N, Costa JJ, Gaspar AR, Santos P (2013) Review of passive PCM latent heat thermal energy storage systems towards buildings' energy efficiency. Energy Build 59:82-103.
- Ahmad M, Bontemps A, Salle H, Quenard D (2006) Thermal testing and numeric simulation of a prototype cell using light wallboards coupling vacuum isolation panels and phase change material. Energy and Buildings 38: 673–681.
- Xu X, Zhang Y, Lin K, Di H, Yang R (2005) Modeling and simulation on the thermal performance of shape-stabilized phase change material floor used in passive solar buildings. Energy Build 37: 1084–1091.
- Solomon AD (1979) An easily computable solution to a two-phase stefan problem. Sol Energy 23: 525–528.
- Tomlinson JJ, Heberle D (1990) Analysis of wallboard containing a phase change material. In: Proceeding of the 25th Intersociety Energy Conversion Engineering Conference, Reno NV, USA.
- Kedl RJ (1990) Conventional wallboard with latent heat storage for passive solar applications. 25th Intersociety Energy Conversion Engineering Conference Reno, NV. pp: 12–17.
- Stovall TK, Tomlinson JJ (1995) What are the potential benefits of including latent storage in common wallboard?. J Sol Energy Eng 117: 318-325.
- Kissock KJ, Hannig JM, Thomas IW (1998) Testing and simulation of phase change wallboard for thermal storage in buildings. In: Morehouse, Hogan (eds). Proceedings of 1998 international solar energy conference, June 14–17, Albuquerque, N. M. USA, American Society of Mechanical Engineers, New York.
- Athienitis AK, Liu C, Hawes D, Banu D, Feldman D (1997) Investigation of the thermal performance of a passive solar test-room with wall latent heat storage. Build Environ 32:405–410.
- Hawlander M, Uddin M, Zhu H (2002) Encapsulated phase change materials for thermal energy storage: Experiments and simulation. Int J Energy Res 26: 159–171.
- Darkwa K, Callaghan PWO (2005) Simulation of phase change drywalls in a passive solar building. Appl Therm Eng 26:853–858.
- Schossig P, Henning HM, Gschwander S, Haussmann T (2005) Micro-encapsulated phase-change materials integrated into construction materials. Sol Energy Mater Sol Cells 89: 297–306.
- Kendrick C, Walliman N (2007) Removing unwanted heat in lightweight buildings using phase change materials in building components: Simulation modelling for PCM plasterboard. Archit Sci Rev 50: 265–273.
- Kuznik F, Virgone J, Noel J (2007) Optimization of a phase change material wallboard for building use. Appl Therm Eng 28: 1291–1298.
- Virgone J, Noël J, Reisdorf R (2009) Numerical study of the influence of the thickness and melting point on the effectiveness of phase change materials: application to the renovation of a low inertia school. In: Proceedings of the 11th international IBPSA conference. Glasgow, Scotland.
- Constantinescu M, Anghel EA, Buixaderas E, Pavel PM, Popa VT (2013) Shape-stabilized nano composite elements based on Phase Change Materials (PCM)-Epoxy for hauses with low energy consumption. In: Proceedings of the 2nd international conference on sustainable energy storage in buildings, Trinity College, Dublin, Ireland.
- Rozanna D, Salmiah A, Chuah TG, Medyan R, Thomas Choong, et al. (2005) A study on thermal characteristics of Phase Change Material (PCM) in gypsum board for building application. J Oil Palm Res 17:41–46.
- Riza M (2007) Thermal characteristics of eutectic mixture of capric-lauric acids as Phase Change Material (PCM) in gypsum board. Jurnal Teknologi Proses 6: 31–38.
- Kośny J, Yarbrough DW, Riazzi T, Leuthold D, Smith JB, Bianchi M (2009) Development and testing of ignition resistant microencapsulated phase change material. In: Proceedings of the Effstock 2009 the 11th international conference on thermal energy storage, Stockholm, Sweden.
- Dhanusiya G, Rajakumar S (2013) Thermal analysis of PCM based building wall for cooling. Int J Sci Eng Technol Res (IJSETR) 2.
- TESS (2016) Thermal Energy Systems Specialists LLC-TESS Libraries- indivisual component libraries.
- Castell AL, Martorell I, Medrano M, Pérez G, Cabeza LF (2010) Experimental study of using PCM in brick constructive solutions for passive cooling. Energy Build 42: 534–540.
- Ibanez M, Lazaro A, Zalba B, Cabeza LF(2005) An approach to the simulation of PCMs in building application using TRNSYS. Appl Therm Eng 25 : 1796-1807.
- Khudhair AM, Farid M (2004) A review on energy conservation in building applications with thermal storage by latent heat using phase change materials. Energy Convers Manag 45: 263–275.
- Xu X, Zhang Y, Lin K, Di H, Yang R (2005) Modeling and simulation on the thermal performance of shape-stabilized phase change material floor used in passive solar buildings. Energy Build 37: 1084-1091.
- Ahmad M, Bontemps A, Salle´e H, Quenard D (2006) Thermal testing and numerical simulation of a prototype cell using light
- Latent heat storage in building materials.
- Peippo K, Kauranen P, Lund PD (1991) A multicomponent PCM wall optimized for passive solar heating. Energy Build 17: 259-270.