Case Report, J Nanomater Mol Nanotechnol Vol: 12 Issue: 1
Nano Science and Nanotechnology Stand in Good Stead against the Current Infectious Disease COVID-19
Vijay Singh1*, Mahavir Singh1, Khalid Mujasam Batoo2 and Syed Farooq Adil3
1Department of Physics, Himachal Pradesh University, Shimla, India
2Department of Chemistry, King Abdullah Institute for Nanotechnology, Saudi Arabia
3Department of Chemistry, King Saud University, Riyadh, Saudi Arabia
*Corresponding Author: Vijay Singh
Department of Physics, Himachal Pradesh University, Shimla, India
Tel: 7559710910; E-mail: singhvj1793@gmail.com
Received date: 10 January, 2023, Manuscript No. JNMN-23-19248;
Editor assigned date: 12 January, 2023, PreQC No. JNMN-23-19248 (PQ);
Reviewed date: 26 January, 2023, QC No. JNMN-23-19248;
Revised date: 02 February, 2023, Manuscript No. JNMN-23-19248 (R);
Published date: 09 February, 2023, DOI: 10.4172/2324-8777.1000351
Citation: Singh V, Singh M, Batoo KM, Adil SF (2023) Nano Science and Nanotechnology Stand in Good Stead against the Current Infectious Disease COVID-19. J Nanomater Mol Nanotechnol 12:1.
Abstract
Infectious diseases are a disorder caused by pathogenic microbes, such as bacteria, viruses, fungi, and parasites. The current “COVID-19” is a viral one, before this, 21st century has witnessed diverse other communicable viral diseases ranging from HIV/AIDS, Ebola Virus Disease (EVD), Avian Influenza (AI), Zika Virus (ZIKV), to two siblings of the COVID-19 causative virus (SARS-CoV-2) named severe acute respiratory syndrome coronavirus (SARS-CoV-1), and Middle East Respiratory Syndrome (MERS), shattering many lives. After the first case which was reported back on 8th December of the last year, the ongoing pandemic has claimed millions of life worldwide and has drastic effect on the global economy.
Keywords: Nano science, Nanotechnology, Respiratory syndrome, Pathogenic microbes
Abstract
Infectious diseases are a disorder caused by pathogenic microbes, such as bacteria, viruses, fungi, and parasites. The current “COVID-19” is a viral one, before this, 21st century has witnessed diverse other communicable viral diseases ranging from HIV/AIDS, Ebola Virus Disease (EVD), Avian Influenza (AI), Zika Virus (ZIKV), to two siblings of the COVID-19 causative virus (SARS-CoV-2) named severe acute respiratory syndrome coronavirus (SARS-CoV-1), and Middle East Respiratory Syndrome (MERS), shattering many lives. After the first case which was reported back on 8th December of the last year, the ongoing pandemic has claimed millions of life worldwide and has drastic effect on the global economy.
Keywords
Nano science; Nanotechnology; Respiratory syndrome; Pathogenic microbes
Introduction
Thus, becoming a major focus of political and scientific attention. Scientific community has been putting their best to find out the best possible solution to control and eradicate COVID-19 [1]. Among the different approaches to fight and terminate current CoV, nanotechnology can significantly combat COVID-19. NT can provide tools which could survey the body from inside and could last longer in the body, even on respiration monitors which are very sensitive. Nanotools can be used to detect, treat, and mitigate the spread of COVID-19. Some important mentioning illustrated [2].
Case Presentation
Nanotechnology in a broader sense comprised of inorganic, organic, lipid based, self assembled protein, and Virus like (VLNPs) nanoparticles that are of utmost significance attributing to their unusual physicochemical properties arise due to larger surface area to volume ratio, and quantum confinement effect at the nano-regime (especially, 1 nm-100 nm) [3]. NPs uphold prospect beyond imaginations in CoV treatment because these are dimensionally comparable to SARS-CoV-2. Due to their nano dimensionality these particles could easily interact or bind with SARS-CoV-2 proteins leading to the disruption of its structure. These have been employed for decades as vectors in specific targeting of drugs, anti-microbial agents and so on (Figure 1) [4].
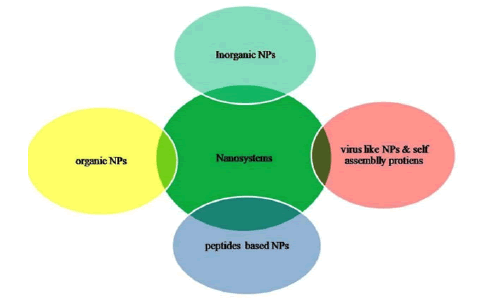
Figure 1: Nano-tools to strike COVID-19 virus.
Besides nano-dimensions, nanoparticle surfaces could be modified and functionalized further. Thus, could bind with ease to spike proteins at S1 or penetrate into the envelope, nucleocapsid and rupture RNA [5]. NPs could flow in the bloodstream without any possible hindrance or without disrupting other functions, especially the one in the human immune system. In this ongoing rough period NT can offer better diagnostic kits, anti-viral nanomedicines, anti-viral coating surfaces, nano masks and even the nanovaccines and nanovectors for therapeutic or vaccine delivery at specific location in the body (Figure 2) [6].
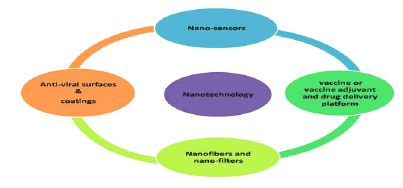
Figure 2: Applications of nanotechnology to combat COVID-19.
The extraordinary characteristics which make NPs so momentous also make them a concern for the United State Food and Drug Administration (FDA) [7]. NPs-biological matter interaction alters the surface characteristics of NPs, depending on their environment which in some cases lead to toxic effects. But, there are some FDA approved NPs belonging to organic, inorganic, lipid, self-assembled protein, and VLNPs classes. Researchers have achieved landmark by understanding the structural, functional and pathological characteristics of SARS-CoV-2 as this would pave the way to design vaccines and therapeutics. Herein, just the glimpse of SARS-COV-2 has been covered [8].
SARS-CoV-2
Novel Coronavirus (nCoV) is a strain from a virus family called ‘coronavirus’. CoVs are from the family coronaviridae and the order nidovirales, classified into four genera: Alphacoronavirus, betacoronavirus, gammacoronavirus, and deltacoronavirus [9]. From various studies researchers have learnt that this virus is in close relation with previously studied CoV, SARS-CoV-1 i.e. both share approximately 76% amino acid identity. Like SARS-CoV-1, SARSCoV- 2 too strongly adhere to Angiotensin Converting Enzyme 2 (ACE2) which is an enzyme molecules found in cell surface and even this binding percentage is quite higher [10]. SARS-CoV-2 virions are in spherical or oval form with the average diameter in nanoscale (approx. 125 nm) and comprised of different lipid viral envelopes and with the positive single stranded RNA genome. Main viral membranes are the Spike protein (S), the Envelope (E), the Membrane (M), and the Nucleocapsid (N) (Figure 3) [11]. S envelope has dual functions of protecting the virus outside the cell and letting it to enter the target cell. SARS-CoV-2 is inactive outside the target cell and only gets active when enter the cell. Viral entry is a complex process which happens via two mechanisms, in first viral spike glycoprotein sub-unit S1 cleave rapidly with ACE2 allowing its entry into the healthy human cell (lock and key mechanism) and in second the virus priming takes place by trans-membrane protease serine (TRMSS2), fusing viral cell membrane with target cell membrane (Figure 3) [12].
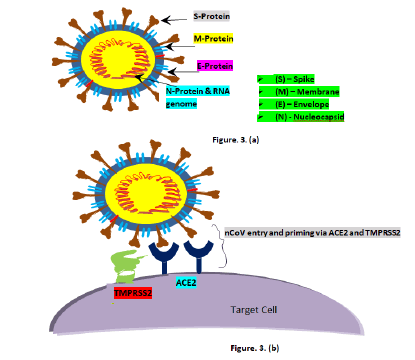
Figure 3: a): Schematic diagram of SARS-CoV-2; b): Schematic diagram of viral entry and priming by ACE2 and TMPRSS2 respectively.
Unlike SARS-CoV-1, SARS-CoV-2 shows furin cleavage site at both the S1 and S2 units boundary providing upper hand in human to human transmission compared to other CoVs. Once the virus penetrates into target cell it releases its genetic material (RNA), which then replicates i.e., use host cell nucleus to create new viral RNA particles. After successful biosynthesis, new viral RNA particles assemble and released. If these are not terminated by the immune system then they continue to replicate [13].
Results and Discussion
The current virus infects the respiratory system, especially the lung epithelium, and has high transmittable rate among humans, even at its dormant stage. Studies indicated that the novel virus essentially transmits through respiratory droplets and contact modes. Currently, no solid evidences are there indicating airborne transmission on broader scale. This transmission is only seen to happen in close and crowed places like health care centres. In principle airborne transmission is different from droplet transmission as former occur by droplet nuclei, and later by respiratory droplet. Depending on the droplet particle size, particle size with >5 μm-10 μm diameter referred as respiratory droplet and those with <5 μm as droplet nuclei. Once the virus is released from the host cell, to infect a person it needed to reach and unload from the vesicles, analogous to what happens in nanomedical membrane vesicle [14]. Thereby it can be expected that various techniques developed for highly active nanoparticles dispersed in aquatic solutions also work for nCoV. SARS-CoV-2 is both symptomatic and asymptomatic. Asymptomatic nature of the virus is of concern as it could triggered community spread causing chaotic situations. Effects of COVID-19 have seen to be different for different people. Common symptoms are dry cough, tiredness, and fever, in addition to a few others are headache, sore throats, loss of taste and smell, a rash on skin, or discoloration of fingers or toes, diarrhoea, and whereas serious one reported includes chest pain, shortness of breathing, or even loss of speech and movement . Average period of incubation reported for COVID-19 is of 4 to 5 days mostly affecting children, elders of age above 60-65 years, and, those already suffering from chronic diseases, whereas the mortality rate of SARS-CoV-2 is found to be very lower than its sibling. Researches done on survival of SARS-CoV-2 over different surfaces revealed that the survival rate of it is similar to that of SARS-CoV-1. Found more stable at smooth surfaces, plastic and stainless steel (3 to 7 days), copper upto 4 hrs, paper and glass upto 4 days, cardboard upto 24 hrs and on wood upto 2 days., and also found stable in a wide range of pH values at ambient temperature (pH 3-10). Cu found effective against viral stability, attributed to Cu-ion release and Reactive Oxygen Species (ROS).
Estimated life span of the virus at different surfaces in comparison to SARS-CoV-1 has shown in Table 1. Recent study has shown, the surface stability of the current virus does not showcase strong variation at 4°C, room temperature and even at 30°C. According to the researchers, while conducting lab testing the human and the environmental factors like viral load, humidity, and solar radiation were not taken into account [15].
Table 1: Aerosol and surface stability of SARS-CoV-2 in comparison to SARS-CoV-1.
Proposed strategies against COVID-19
To contain and mitigate SARS-CoV-2 different steps have been taken by WHO, governments, and scientific community. WHO has established “Solidarity” an international mega clinical trial, aimed to check rapidly discovered drugs, whether they slow disease or improve survival. Over 100 countries have joined the trail and many have shown interest. Different ways to combat COVID-19, foremost slowing or preventing virus spread and transmission using chemical sanitization such as, <60% ethanol, sodium hypochlorite, iodine, and quaternary ammonium salt disinfectants, hydrogen peroxide (H2O2), other by using antiviral surface coating/self sanitizing surfaces, particularly to mitigate fomite transmission and wearing masks.
Thereafter, early diagnosis and followed by adequate medication. Upto date, no well-defined drug is viable for COVID-19 and hospitals being overwhelmed with COVID patients. Thus, seeing the desperate need of treatment scientist have suggested various drug combos, but only four has been preferred and recently one more got added into the list.
Mention in Table 2, with their respective action mechanism.
Table 2: Drugs used against COVID-19.
Race is on to find specific cure/vaccine, whereas in the meantime researches and public health agencies are going for repurposing already approved drugs which are largely safe. In addition to this they too are looking for drugs which have shown effective against the SARS and MERS outbreaks or even in animal trials. Drugs with most likelihood of working are remdesivir, hydroxychloroquine, ritonavir/ lopinavir, ritonavir/lopinavir-interferon-beta-1a, and dexamethasone.
Out of which the use of rest are revoked by FDA and WHO. Till date only the two are found most effective one steroid (dexamethone) and other one is antiviral (remdesivir). Besides, these there are many diverse strategies in use to fight COVID-19 such as convalescent plasma therapy and so on. The two most successful remdesivir and dexamethasone are found effective only in the last stage and no report is there indicating effectiveness of the drug in mild cases. Moreover these conventional strategies have their own adverse effects depending on the dose, duration, and frequency of administration to COVID patients). Thus, there is massive need of new strategies which can overcome the drawbacks of conventional strategies and prove to be more efficient. Nanotechnology based strategies could serve the purpose and eventually they are doing too.
Nano tools and strategies against COVID-19
Inorganic Nanoparticles (Ing.NPs): Metallic Nanoparticles (mNPs) have shown strong efficacy against viral infections. The SARS-CoV-2 found to have a very shorter life time for copper which is in close agreement with SARS-CoV-1. Same is the case with copper alloy surfaces where for the Cu% is linear with the inactivation of CoV, which is attributed to Cu-ions released and ROS (Reactive Oxygen Species) yielded. Silver (Ag) NPs and Gold (Au) NPs also exhibit broad spectrum of antiviral effects. Moreover the structural and surface morphology of NPs have a significant role in deciding their antiviral efficacy and their embedment in polymer matrices could enhance the chemical stability and antiviral efficacy, binding efficiency to targeted sites, controlled release and also minimizing their release into the environment.
The antiviral action of mNPs, evidently of AgNPs and AuNPs are well studied. These NPs have shown low toxicity to humans and their antiviral action could be accelerated by coating with polymers and toxicity could be minimized using biocompatible polymers particularly those approved by the FDA. Against HIV-1, AgNPs interacted with cell receptors prohibit the entry of virus into the host cells. AgNPs inhibited viral replication by interacting with viral genome in case of double strain RNA (dsRNA) viruses. AgNPs used against H7N3 a subtype of avian influenza A virus, to stop viral entry and viral replication. Silver NPs functionalized with tannic acid and mercaptoethane-sulfonate found effective against HSV infection through direct inhibiting viral attachment, viral penetration and postinfection spread. AgNPs clinched on textile fabrics against influenza- A and Feline Calicivirus interact with viral envelope and impaired them, thus, resulting in viral inhibition. Similarly, AuNPs coated with biocompatible polymers have also found to exhibit significant antiviral efficacy against HIV-1 and a few influenza viruses (e.g. H1N1, H3N2, and H5N1) respectively. Recently, a study indicated, AuNPs as antigen carrier and an adjuvant in immunization, inducing strong IgG response but not succeeded in improving vaccine efficiency this attributed to high allergic inflammatory responses, the same study also revealed, Toll Like Receptor (TLR) agonists adjuvant vaccine induced strongly protective antibodies without eosinophilic infiltrations. In another recent study, anti-viral action of Porous-AuNPs (PoAuNPs) against influenza-A virus was elucidated. Herein, the magnitude of antiviral activity was found in the decreasing order PoAuNPs> AgNPs>solid AuNPs (almost zero), the attenuated infectivity of influenza-A virus for PoAuNPs ascribed to its extensive specific area, better stability under saline conditions and stronger affinity for Hemagglutinin (HA) an effective antiviral target sites. Other study, revealed that sulfated ligands coated AuNPs, AgNPs, and hybrid Ag-Cu NPs could suitably bind to HIV gp120 (glycoprotein) and could significantly inhibit in vitro HIV-1 infection in cellular models. Further, study has also demonstrated a sizedependent (size range, 1 nm-10 nm) interaction between NPs and HIV-1. In one study, researchers developed a novel Aunanorod based peptide inhibitor, which selectively target S protein of MERS and this novel antiviral agent was found biocompatible and biostable in both in vitro and in vivo studies. A research demonstrated, SARS-CoV-1 along with some other microbes was when exposed to metal catalysts Ag/Al2O3 and Cu/Al2O3 surfaces effectively attenuated infectivity of SARS-CoV-1 and other microbes used and more promising results got when metal catalysts were exposed to air. Thus, these could be competent enough to use as air disinfectants at places with high risk zone like hospitals, public transport and households, the inactivation of microbes attributed to catalytic oxidation in lieu of toxicity of heavy metals. In recent years, along with CuNPs, AgNPs, and AuNPs, because of low toxicity and brilliant activity selenium NPs (SeNPs) antiviral potentiality too have become popularized among the nanotechnology community. A study showed, increased capability of Oseltamivir (OTV) functionalized SeNPs to prevent HINI infection, antiviral action of Se-OTV nanosytem attributed to the release of Se-species exhibiting antiviral properties against multidrug resistance.
Metal Oxide Nanoparticles (MO-NPs) too have found very promising in destroying virus, particular focus is on magnetic oxide (magnetite), CuO, ZnO, and titanium oxide TiO2. A study conducted on antimicrobial activity of polyurethane/CuO nanocomposite projected it as an effective antimicrobial filter for air purification. In a recent study, SiO2-Ag NPs are used as an active material against MS2 bacteriophage showing their possible application as an antiviral air filter system in transportation. Zinc Oxide (ZnO) being an important ingredient of many cosmetics, enzyme, and ointments for pain and itch relief looks promising in the current fight. ZnO antimicrobial properties are known from the time long past and recent studies too revealed the same. Study done on ZnO Tetrapods (ZnOTs) against Herpse Simplex Virus-2 (HSV-2), demonstrated ZnOTs capability in neutralizing the virus virions and stopping the virus entering the cell. Thus, making ZnOTs a suitable candidate for HSV-2 antiviral therapy. ZnO functionalized Polyethylene glycol nanosystem has found effective for inhibiting HSV-1. ZnO NPs along with Zn salts have proven to be very efficient antiviral agent with minimal toxicity.
agent with minimal toxicity. Similar to ZnO, and CuO, TiO2 can also emerge fruitful due the photocatalytic reaction mechanism. These materials might also used to design antiviral coatings particularly stimuli responsive based on photocatalytic, photothermal, or electrothermal treatments against SARS-CoV-2.
Magnetic NPs (MNPs) particularly Iron Oxides (Fe3O4) after being approved by FDA sounds good candidate especially as nanocarriers for vaccine and EUA antiviral-drugs. Thereby, lessening adverse side effects of EUA drugs. MNPs can also be highly significant in developing simple and cost effective detection protocols for nCoV.
While using magnetic NPs their extraordinary magnetic properties, possibility of further surface modification and organic ligand functionalization is of utmost significance (Figure 4).
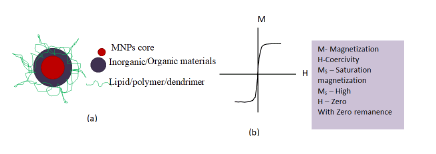
Figure 4: a) Schematic representation of multifunctional magnetic nanoparticles; b) Schematic representation M-H curve with superparamagnetism.
The nanosystem can be easily controlled due to their magnetic character attributed superparamagnetic behaviour. Fe3O4 antiviral activities have been investigated to a greater extend in recent years. A study indicated CuFeO2 crystals having rhombohedral like structure with well-defined edges, corners, and smooth surfaces as a novel antimicrobial material when studied against influenza virus using bacteriophage Qβ model. Various studies have indicated, Fe3O4 NPs also exhibit excellent antiviral efficacy essentially at lower dose. The Fe3O4 nanozyme have shown significant broad spectrum of antiviral efficacy against the 12 subtypes of influenza-A virus. In addition to this, Fe3O4-nanozymes when applied to facemask has shown to increase viral protection ability of the mask against H1N1, H5N1, and H9N7 subtypes of influenza virus which uses to possess serious threat to human health. Here to inactivate the Virus, lipid peroxidation present in the lipid envelope of the virus subtypes has been catalyzed by Fe3O4-nanozymes. Moreover, other NPs like graphene and its derivatives Graphene Oxides (GO) and reduced Graphene Oxides (rGO), Quantum Dots (QDs), Carbon Nanotubes (CNTs), and nano diamonds too have better antimicrobial properties and can be a better weapon against COVID-19. Ing-NPs reported to behold better antiviral efficacy attributed to ROS (surface oxidation), photoreaction and photo-thermal properties (Figure 5).
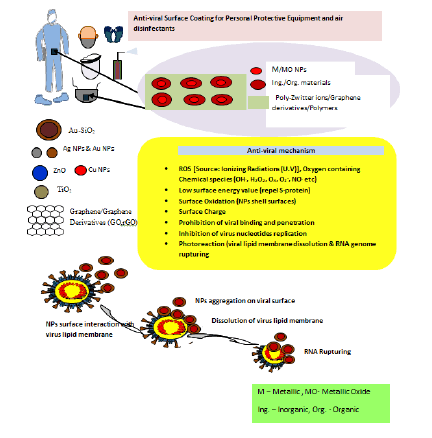
Figure 5: Nano based disinfectants for protective equipments, air, and surfaces. Followed by schematic representation for the antiviral mechanism of nano based surface coating.
This prevents the virus transmission by prohibiting it from binding and penetrating into the host cell. Besides, inorganic NPs particularly Au NPs, Graphene Sheets (GS), QDs, CNTs, GO, rGO, and MNPs can be of tremendous usage for rapid and accurate detection of the SARSCoV- 2. The nanosensor/biosensors being developed using these NPs could be further advanced or modified by coating the Ing-NPs with biological entities like antibodies etc. for better binding with the glycoproteins of SARS-CoV-2. Thereby, enhancing the efficiency of sensing devices. Although the toxic behaviour of these metallic and metallic oxide NPs lessons the hope for their usage but it is retained by surfacely modifying or embedding them in the organic-NPs (polymeric, lipid, dendrimers, etc.) and this is when organic-NPs come into the picture.
Organic nanoparticles
Polymeric nanoparticles: This class of NPs comprised of synthetic and biopolymers. Based on their monomers and multi-functional groups Polymeric-NPs have been frequently used in surface modification of inorganic NPs, medical adhesives, sealants, and in target drug delivery. For better efficiency, these polymers are bridged with each other through cross-linkers either by ionic or covalent bonds forming a 3-D structure. Some chronically used polymeric-NPs polyurethane, Polyvinyl Alcohol (PVA), Polyethylene Glycol (PEG), chitosan, Polyglycolic Acid (PGA), cellulose, aliginate, gelatin, collagen, and so on. The hydrophilic polymers such as PEG (most famous ones) used in surface modification of NPs. Thus enhance the time duration of NPs inside the body. In addition, PEG has the potential to lessen NPs interaction with digestive enzymes mucus, thereby protecting nanosystems. Chitosan (cationic polysaccharides) NPs have shown strong affinity for lung epithelial tissues. These are biocompatible, biodegradable, and have broad antimicrobial spectrum.
The biodegradability of the chitosan-NPs is proportional to their degree of deacetylation. Current studies indicated that chitosan can be a worthy tool against COVID-19. For instance, Novochizol™, chitosan-NPs based drug delivery system can act as suitable cargo for anti-COVID-19 drugs. The best part of the polymeric-NPs is that many of them are FDA approved. Some of the FDA approved polymer NPs applied in medicine and pharmaceuticals; Polylactides (PLA), PGA, PVA, Poly Lactide-Co-Glycolides (PLGA), and PEG. PLGA NPs, based nanovaccine created by researcher have found potent in delivering STING agonists and subunit viral antigen to the immune cells and lymph nodes. Copolymer NPs used exhibited pH responsive release profile with advantages like clear local activation, and lessened systematic reactogenecity (common side effects after vaccinations).
Thereby, polymeric-NPs uphold a great potential alone or in nanocomposite form with Ing-NPs in mitigating SARS-CoV-2 dissemination and eradication.
Lipid Nanoparticles (LNPs): Due to excellent biocompatibility, long term stability, easier synthesis protocol, and application versatility lipid based nanoparticles have shown promising results in biomedical field. Among numerous LNPs, liposome are widely studied and used by researchers. Liposomes have excellent bioavailability and excellent biodegradability, improved capability to stabilize therapeutic compounds, enhance cellular and tissue uptake efficiency, and efficient biodistribution of compounds at specific sites. Moreover, after the approval of particularly Doxil® by FDA and successful clinical trials of many liposome based systems, liposome nanoparticles, these particles got much attention. Liposomes are spherical vesicular systems with atleast one lipid layer where phospholipid is the main gradient in liposome. Liposome varies in size ranging from ~20 nm to 100 nm (in tiny unilamellar vesicles), 100 nm to 3000 nm (in large unilamellar vesicles) and >3000 nm to a few μm (in multilamellar vesicles). Liposomes can be loaded with both hydrophilic and hydrophobic therapeutic agents in a single vesicle.
Various studies have indicated successful use of liposome and its derivatives in treating infectious diseases, particularly for which viruses are responsible. For instance, optimized release of an effective antiviral therapeutic agent named N-Butyl-Deoxy-Nojirimycin (NBDNJ), against viral infections like Human Immunodeficiency Virus (HIV) and hepatitis viruses via liposome. This not only lessen the effective dose of an therapeutic agent required for antiviral activity, but may also improve the absolute anti-viral effect as a consequence of attacking the virus via multiple mechanisms. A study conducted on mice has shown that surface linked liposomal peptide could be a useful candidate for cytotoxic tlymphocytes based vaccine against SARS-CoV-1. Recently, it was purposed that ACE2 and liposome can be fused together to a form a complex called “pulmonaryproteoliposomes” which would act as bait for SARS-CoV-2, thereby inactivating it. According to the authors their purposed strategy against current virus could lessen the total viral load of pulmonary tissues, thus serving immune system to successfully ward off viral infection. As therapeutic agent cargos, liposome has some downside such as low in vitro and in vivo stability, low encapsulation efficiency, high fabrication cost, early removal from blood and capture by RES cells. At current, LNPs are in usage as a candidate vaccines for COVID-19 for instance; mRNA 1273 of Mondera TX. Inc., which at the 3rd stage of clinical studies with registered no. NCT04470427. 3 LNP-mRNAs (BNT162) of BioTech SE Pfizer again in phase III of clinical trials with registered no. NCT04368728. And some are in preclinical trials in the section of nanovaccine/vaccine adjuvants.
Niosomes: Niosomes are non-ionic surfactant vesicular delivery systems, structurally analogous to liposomes and could deliver both hydrophilic and hydrophobic drugs. Niosomes have advantages over liposomes like easy and cheap to synthesize, exhibit good stability, high biodegradability, good biocompatibility, and low toxicity. The key components of niosomes are squalene (natural lipid), polysorbate (non-ionic surfactant) and so on. To achieve better stability adequate amount of cholesterol is added to the noisome. Niosomes offer an optimistic approach, a novel drug carrier. Due the benefits of niosomes over liposomes researchers should explore these NPs for the application against COVID-19.
Dendrimer nanoparticles: Dendrimers are hyperbranched 3- dimension tree like nanostructures; these are comprised of three major parts, a symmetrical core, an inner, and an outer shell, each with varying characteristics such as solubility or binding with the ligands.
In general, dendrimers size is below 100 nm. Nanometric dimension and polymeric branches make these particles potential candidates against COVID-19. Due to their significant biological and chemical properties dendrimers found useful in DNA, siRNA and antiviral therapeutics delivery. Further, their properties can be modified by employing diverse polymers and functional groups. Functional groups of these NPs could terminate virus by interacting with the cell surfaces. Dendrimers can also be used to detect SARS-CoV-2, enhance solubility of anti-viral drug delivery system and as antiviral component. For example, a study demonstrated inhibitory character of poly (amidoamine) PAMAM-dendrimers when conjugated with either 3’-Sialyllactose (3SL) or 6’-Sialyllactose (6SL) against different range of avian influenza virus strains. According to recent finding SPL7013, a dendrimer showed significant inhibitory activity against SARSCoV- 2, earlier this nano-product has shown strong against HIV and HSV infections. A study conducted on PAMAM-dendrimers against MERS-CoV, demonstrated improved delivery of antivirals and intrinsic antiviral activity.
VLNPs
Usage of VLNPs in biomedical areas has surged in recent years.
VLNPs are usually of the dimension between 20 nm to 200 nm and resembles with virus structures, but devoid of viral genomic materials which make them non-infectious. Thus, increase safety during manufacturing and administration of these particles. VLNPs are widely used and studied to prepare vaccines which are better than conventional vaccines. Nanometric size and potential to mimic the authentic virus or antigen in terms of structure and antigenic determinants make them potent enough for the treatment of viral infections and for viral diagnosis. VLNPs for vaccine development and drug delivery systems can be prepared by diverse methods two of them are surfactant treatment and mechanical extrusion using an extruded. VLNPs were formed from M-protein of SARS-CoV-1 by simultaneous expressions with E or N protein in insect cells and mammalian cells. Similarly, VLNPs of MERS-CoV were developed by co-expression of E, S and M proteins in insect cells, which can be used for designing vaccines. Researchers developed S proteindisplaying nanovesicles using silkworm larvae and Bm5 cells in order to develop nano-based vaccines and diagnostic process against MERSCoV. Insect larva and insect cells have been well established hosts for the productions of VLNPs. Due to the potential to induce more immune response VLNPS are in higher utilization against SARSCoV- 2 to develop vaccine of the section nanovaccine/vaccine adjuvants.
Self-assembling Protein Nanoparticles (SAPNs)
These noble nanoparticles are prepared from the oligomerization of monomeric proteins with a diameter ranging from 20 nm-100 nm.
These nanoparticles are synthesized from a single protein building block through spontaneous self-assembling into a stable 3D nanoparticulate system, promising a highly versatile vaccine platform. SAPNs found promising in the fight against viral diseases such as SARS, influenza, HCV, and HIV. 1c-SApNP is a single component SAPN based vaccine platform technology which has found promising in its initial studies and that made the ufovax vaccine company to extend this technology to a vaccine against COVID-19, this platform has also found promising against HCV.
Nanoparticles in SARS-CoV-2 detection
Accurate and rapid diagnostic and surveillance is needed for containing the virus spread. At present current diagnostic techniques are; Reverse Polymerase Chain Reaction (RT-PCR), gene sequencing, a lateral flow immunoassay, a common Point-of-Care (POC) diagnostic approach which detects antibodies against current virus in patient samples, and, chest Computed Tomography (CT). Many diagnostic kits contain three assays, each of these target a different gene present in the virus like Orf1 gene (human RNA polymerase protein), N-gene (the nucleocapsid protein) and E-gene (envelope protein) respectively and only a few limited ones use to target the Sgene (spike protein). Convention techniques have some drawbacks which should be addressed for better and early diagnosis of COVID patients. Nanostructure materials based biosensing kits are solid candidates for the work.
Materials in their respective nano-size exhibit tremendous properties such as surface Plasmon effect, large actives sites for binding, enhanced optical, electrical, and magnetic properties that provide a platform for next generation viral diagnosis process. Sensors fabricated using nanomaterials are termed as nanosensors. Advantages of using nanosensor technologies over other detection methods are possibility of early stage detection, and management of targeted diseases due to their excellent sensitivity, and specificity, possibility of measuring at the single molecule level. Besides, nanosensors are cost effective, have quick response time, more stability and allow multiplexing. Nanosensors, used to sense biologically specific materials termed as nano-biosensors.
American biochemist L.L Clark, for the very first time introduced biosensor to the scientific communities in the year 1956, which was used for oxygen detection in the blood, and the electrode used herein was named ‘Clark electrode’. Since then this field has progressed a lot and after the fabrication of nanoparticles based biosensors this field has shown a broad spectrum of applications particularly in biomedical field. Nanobiosensors have shown a tremendous potential in detecting infectious diseases. Thus, these can contribute a lot in the fight against COVID-19. In norwegian university of science and technology, researchers developed a testing kit using silica decorated Fe3O4, this kit was used to test around 1,50,000 COVID-19 patients. Herein, silica exhibit strong affinity for RNA genome of SARS-CoV-2. Later, the binding system was separated magnetically and submitted for RTPCR. (nanowerk.2020). A group of researchers developed an optical sensor aiming to detect SARS-CoV-2 concentration in the air. In principle, the nanostructured biosensor works on the both optical effect (Local Surface Plasmon Resonance, LSPR) and thermal effect (Plasmonic Photothermal, PPT) simultaneously. In this sensor the surface functionalized (with complementary DNA receptor) 2D-tiny gold structures, “Gold Nanoislands” (AuNI) plasmonic chips were used in LSPR systems for specific viral sequence detection and the grafted DNA receptors analogous to the specific RNA sequences of the nCoV are used.
An ultrabright fluorescent nanoprobe “plasmonic Fluor” developed for SARS-CoV-2, where Au NPs behave as beacons of light. According to authors, this nanoprobe is 100 times more efficient than conventional sensors and can detect tiny amounts of target biomolecules present in biofluids and even those present on the cells.
Recently, researchers have reported a protocol in which the viral RNA is taken from different specimens using automation process, assisted by multifunctional magnetic spinel nanoferrite. Here, ZnFe2O4 is firstly coated with silica and then further functionalized with carboxyl modified PVA. According to the researchers this is a simple and cost effective protocol which might aid to reduce operation time and is more efficient than the conventional approaches for current molecularlevel diagnostic of COVID-19. In other published research, researchers have developed a biosensor which can visually detect SARS-CoV-2 in 10 minutes of time, without the need of any advance laboratory techniques. The reported biosensor is a simple calorimetric assay containing thiol modified Antisense Oligonucleotides (ASO) in association with anisotropic plasmonic nanosized Au particles. In this bioassay two separate regions of the N-gene of the current virus are being specifically targeted by ASO, and Au NPs are used to detect the alternation in colour when the virus is present. In addition, the designed bioassay could also be modified to target other regions of the viral genome. At the korea basic science institute, researchers fabricated a biosensing device based on GS of the ultrasensitive-Field Effect Transistor (FET) which can detect S-glycoprotein of SARSCOVID-CoV-2. Here, a specific antibody is being coated with the GS of the FET. It could detect the S-protein of SARS-CoV-2 present in clinical transport medium at a concentration of 1.31 × 10-3 pM and 1.31 × 10-2 pM when present in buffer saline medium. The best thing about this FET biosensor is that no significant measurable cross reactivity observed with MERS antigen. Thus, it can easily differentiate between the antigens of MERS and SARS-CoV-2 (Table 3).
2020-001072-15
2020-001072-15 ISRCTN89951424Corona VacInactivatedSinovac research and development Co., Ltd.SinovacPhase IIINCT04456595Inactivated vaccineInactivatedHenan provincial center for disease control and preventionWUHAN institute of biological products/sinopharmPhase IIIChiCTR2000034780LNP-encapsulated mRNA (mRNA-1273)RNAKaiser Permanente Washington Health Research InstituteModernaTX, Inc.Phase IIINCT044704273 LNP-mRNAs (BNT162)RNAMultiple study sites in Europe and North AmericaBioNTech SE PfizerPhase IIINCT04368728Adenovirus type 5 vector (Ad5-nCoV)Non-Replicating Viral VectorCanSino biological incorporation, Beijing institute of center for vaccinology biotechnology, CanadianCanSino biologicsPhase I, Phase II, Phase IIIChiCTR2000030906 ChiCTR2000031781 https://www.clinicaltrialsarena.com/news/cansino-vaccine-saudi-trial/
Table 3: Vaccine candidates in Phase III trials, mentioned in landscape documents of COVID-19 vaccine candidates (20 August 2020).
Nanovaccine/vaccine adjuvant
The continuous upsize of COVID-19 cases, particularly in the developing countries where situation has worsened, the need for therapeutics and vaccine has risen. Scientists over the globe are working hard to potent treatment and vaccine. The very first vaccine candidate enter the Phase I human clinical trial on March 16, 2020 under NCT04283461 search identifier. According to 20 August, 2020, landscape document drafted by the WHO, 28 vaccine candidates are under clinical evaluation and whereas, 139 are in preclinical evaluation. Some vaccine candidates like Inactivated vaccine, CoronaVac, mRNA-1273, Ad5-nCoV, sponsored by Sinopharm, Sinovac, Moderna, CanSino Biologics, respectively are in phase III trials. The WHO has prepared this landscape document of vaccine candidates against COVID-19 for shedding light on current status of clinical evaluation and regulatory status (Table 4).
Table 4: NPs based COVID-19 candidate vaccines in clinical mentioned in landscape document (WHO), 20 August 2020.
For a decade, a novel generation of vaccine has captivated the interest of scientists, where nanoparticles being employed in double fashioned way; one as nano-cargo for both active or passive vaccine delivery at target sites and second as potent immune simulator for producing strong immune response. Due to the quirks of nanoparticles nanovaccines have advantages over old fashioned viral vaccines.
Problem with conventional vaccines (DNA and RNA) are safety issue many has shown adverse effects, inefficacious interaction between vaccines and immune cells, low in vivo stability, incursive administration, and inadequate storage and transport issues. And the benefits of nanovaccines are: These are highly goal oriented with strong killer instinct, have good stability, controlled release of antigens, regulate immune response type, have higher depot effect, and can penetrate deep into the cell through endocytosis mechanism, high absorption/uptake efficiency for antigens and so on and so forth. NT platform could offer different types of vaccines based on nucleic acid, peptide, VLPs, SAPNs and polymeric NPs and there many nano vaccines which are currently in clinical and pre-clinical trials for COVID-19 (Figure 6).
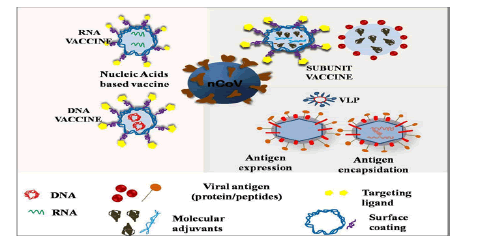
Figure 6: Schematics for nanoparticles based different vaccine platforms.
Those have found effective under in vitro/in vivo applications for SARS-CoV-1, MERS, and other respiratory viruses could play a key role in containing and developing effective vaccine for COVID-19.
A new study that has been published, where researchers, studied 10 COVID-19 patients admitted in ICU (Intensive Care Unit) and indicated that the nCoV-reactive CD4+ helper T-cells are found active in all the patients studied and CD8+ “killer” T-cells are found in 8 out of 10 patients.
Besides, study also revealed that the strongest T-cell response are reported towards the S-protein, and the SARS-CoV-2-specific T cells predominantly produced effecter and Th1, Th2 and Th17 cytokines.
The variation of T-cell responses with the disease severity by T-cell kinetics are reported too. All These studies would play a key role in the development of vaccine against COVID-19 (Table 5).
Table 5: NPs based vaccine candidates in preclinical trials, as per (WHO landscape document 20 August 2020).
The fate of nanovaccines depends on several key factors like size and shape of NPs, charge on the surface, hydrophobicity, and NPs composition. Size and distribution of NPs also play an effective role in the entry of NPs through cell membrane. A study has shown that the optimal size of AuNPs for cellular uptake of HeLa cells is 50 nm.
Besides NPs size is also important in the immune response. For instance, NPs with size <500 nm (particularly 40 to 50 nm), found evoking the responses of the T-cells (CD8+ and CD4+) Type 1 cells, while the NPs with size >500 nm found capable of stimulating T-cells (CD4+) type 2 cells and produce antibodies. Shape of NPs is one of the governing features in the cellular uptake. Study revealed spherical NPs exhibit higher uptake efficiency than non spherical ones. Other factors; the composition and the surface charge of nanoparticles are one of the ruling features in aggregation of nano structured particles in the blood stream, biocompatibility and their circulation time in the body. Thereby, while preparing nanovaccines/vaccine adjuvants for COVID-19 researchers should keep these considerations in mind.
Nano toxicological consideration and future horizons
Despite the potential benefits, the full fledge implementation of nano based products against COVID-19 is still a concern. NPs are highly bioactive especially the metallic ones, after reaching inside the body NPs become systemic based on their environment their surface properties alter, and in some cases such changes result into toxic character of NPs like cytotoxicity, genotoxicity, neurotoxicity, and so on. Potential entry routes of NPs into the body are ingestion, inhalation, dermal, and parenteral. Among various mechanisms behind the nanotoxicity like; activation of Mitogen Activated Protein Kinase (MAPK), damaging of organelles such as mitochondria, lysosomes, nucleus, transforming growth factor β-signaling, damage of cell membrane, DNA, change of cell cycle, release of proinflammatory cytokines, and so on (Figure 7).
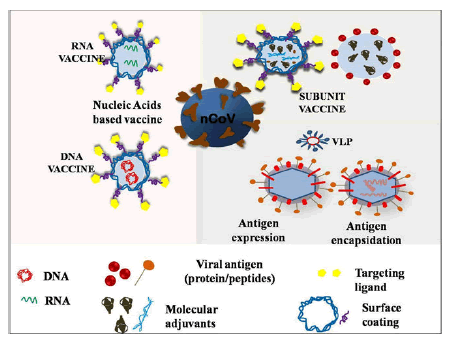
Figure 7: Different mechanisms behind nanoparticles hazards.
The underlying ones are the generation of ROS and the liberation of free radicals, attributed to the high surface area and increased number of particles per unit mass. Various studies have indicated that certain NPs like metallic, metal oxides, CNTs, fullerenes, graphene and its derivatives generate ROS. Major features which are accountable for nanotoxicity are surface chemistry, surface charges, surface area, hydrophobicity, hydrophilicity, shape and size of NPs. Besides, exposure factors like concentration of NPs, duration, and frequency too are responsible for adverse effects of NPs. Thereby, thorough investigation and detailed understanding of biocompatibility and toxicity of NPs are needed for the safe and sustainable development.
Risk assessment of hazards of NPs on human health and environment isn’t much flourished, unlike other vast majority of chemicals there is not set guidelines for the evaluation of hazards behaviour of NPs.
Nanotoxicity assessment is an intimidating task. Because of numerous conflicting nanotoxicological studies, no general consensus has been made for decades which has arisen a question that whether current nanotoxicological testing strategies are worthy enough? There is a strong need of new validated testing approach and advancement in pre-existing nanotoxicity testing strategies. Mathematical and computational methods can play a key role for analysis and interpretation of experimental data, proper predictions of nanoscale systems (deigns and control) seeing the need for the application.
Along with in-vitro/in-vivo, computational modeling more focus should give to epidemiological studies. Nanotechnology, beholds a lot of potential to deal with the pre-existing problems (HIV, HVC), current COVID-19 and futuristic problems similar to these, all issues with its implication should be checked and shorted out as soon as possible to have its full benefit.
Conclusion
As the nanoscience and nanotechnology have progressed, a large number of nanosystems and nanodevices (drug/vaccine carriers, biosensors, etc.) have emerged which slowly but surely is revolutionizing the biomedical field. This clearly showcases the potential that NS and NT have in fighting the current global pandemic. Diverse nanosystems discussed in this review are potent candidates, which can release out the burden from the health care systems and protect them. COVID-19 is neither the first nor the last pandemic which humanity would be facing. Thus, there is strong need for scientists/researchers from multi-disciplines to collaborate and work effortlessly so as to get ourselves equip for current and upcoming situations like this. But before applying them in practical (to market), their toxic behavior should be strongly assessed because every individual’s life is of utmost importance for humanity to progress sustainably. Though, a lot has been done by scientists/researchers and still a lot is needed to be done. The young researchers from NS and NT should understand the importance and excel in these fields to make everything which seem as a dream at present a reality in near future.
Acknowledgement
Author KM Batoo is thankful to the Deanship of Scientific Research at King Saud University for the financial support under the Project Code (RG-1437-030).
References
- Shin MD, Shukla S, Chung (2020) COVID-19 vaccine development and a potential nanomaterial path forward. Nat Nanotechnol 15: 646-655.
[Crossref] [Googlescholar][Indexed]
- Kostarelos K (2020) Nanoscale nights of COVID-19. Nat Nanotechnol 15: 343-344.
[Crossref] [Googlescholar][Indexed]
- Sivasankarapillai VS, Pillai AM, Rahdar A (2020) On facing the SARS-cov-2 (COVID-19) with combination of nanomaterials and medicine: Possible strategies and first challenges. Nanomaterials 10: 852.
[Crossref] [Googlescholar][Indexed]
- Uskokovi V (2020) Why have nanotechnologies been underutilized in the global uprising against the coronavirus pandemic? Nanomed 15.
[Crossref] [Googlescholar][Indexed]
- Talebian S, Wallace GG, Schroeder A, Stellacci F, Conde J (2020) Nanotechnology-based disinfectants and sensors for SARS-CoV-2. Nat Nanotechnol 15: 618-621.
[Crossref] [Googlescholar][Indexed]
- Krug HF, Wick P (2011) Nanotoxicology: An interdisciplinary challenge. Angew Chem Int Ed Engl 50: 1260-1278.
[Crossref] [Googlescholar][Indexed]
- Eifler AC, Thaxton CS (2011) Nanoparticle therapeutics: FDA approval, clinical trials, regulatory pathways, and case study. Methods Mol Biol Methods Mol Biol 726: 325-338.
[Crossref] [Googlescholar][Indexed]
- Sohrabi C, Alsafi Z, O’Neill N (2020) World Health Organization declares global emergency: A review of the 2019 novel coronavirus (COVID-19).
[Crossref] [Googlescholar][Indexed]
- Rabaan AA, Al-Ahmed SH, Haque S (2020) SARS-CoV-2, SARS-CoV, and MERS-COV: A comparative overview. Le Infez. Med 28: 174-184.
- Wang Q, Zhang Y, Wu L (2020) Structural and functional basis of SARS-CoV-2 entry by using human ACE2. Cell 181: 894-904.
[Crossref] [Googlescholar][Indexed]
- Wang L, Wang Y, Ye D, Liu Q (2020) Review of the 2019 novel coronavirus (SARS-CoV-2) based on current evidence. Int J Antimicrob Agents 55: 105948.
[Crossref] [Googlescholar][Indexed]
- Lu R, Zhao X, Li J (2019) Genomic characterisation and epidemiology of 2019 novel coronavirus: Implications for virus origins and receptor binding. Lancet 395: 565-574.
[Crossref] [Googlescholar][Indexed]
- Walls AC, Park YJ, Tortorici MA, Wall A, McGuire AT, et al. (2020) Structure, function, and antigenicity of the SARS-CoV-2 spike glycoprotein. Cell 181: 281-292.
[Crossref] [Googlescholar][Indexed]
- Zhang L, Lin D, Sun X (2020) Crystal structure of SARS-CoV-2 main protease provides a basis for design of improved a-ketoamide inhibitors. Science 368: 409-412.
[Crossref] [Googlescholar][Indexed]
- Shang J, Ye G, Shi K (2020) Structural basis of receptor recognition by SARS-CoV-2. Nature 581: 221-224.
[Crossref] [Googlescholar][Indexed]